Hypertension is categorized as a chronic elevation in blood pressure with a diastolic pressure over 90 mmHg and a systolic pressure over 140 mmHg. Although most hypertension is primary, referring to an increase in blood pressure with no underlying cause, pathologies affecting the kidney or endocrine system can also cause hypertension. This is termed secondary hypertension.
Mechanism of Primary Hypertension
The precise mechanism of primary hypertension has not yet been explained, although dysfunctions in mechanisms regulating vascular tone, both directly and indirectly, have been pinpointed as exerting a significant influence on hypertension. In hypertension, an increase to arterial pressure is identified by specialized mechanoreceptors known as baroreceptors, which occur in the carotid sinuses and the aortic arch. Baroreceptors undergo innervation by nerves which synapse in the nucleus tractus solitarius (NTS), a section in the medulla oblongata that modulates blood pressure via the regulation of sympathetic and parasympathetic transmission. In the event of an increase in blood pressure, the baroreceptor firing rate also increases, thus stimulating the activation of sympathetic neurons originating in the NTS and synapsing in the outer arterial wall, or adventitia.
The activation of these sympathetic neurons instigates vasoconstriction via the release of noradrenaline, in conjunction with the subsequent activation of Gq and the downstream IP3 signal transduction pathway. Consequently, drugs targeting α adrenergic receptors regulate blood pressure. The exact impact to vascular tone depends on the α adrenergic receptor subtype; α1 adrenergic receptors trigger the release of noradrenaline from sympathetic nerve terminals, while α2 adrenergic receptors block the release of noradrenaline, operating as a feedback mechanism to regulate its release from sympathetic nerve terminals.
A Well-Proven Technique in Hypertension
As well as sympathetic mechanisms, targeting the renin-angiotensin-aldosterone system (RAAS) is a well-proven, effectual technique in hypertension. The activation of the RAAS in response to a drop in blood pressure engenders the release of renin from the juxtaglomerular apparatus in the kidney (Figure 1). Renin cleaves angiotensinogen, which undergoes additional cleavage to generate angiotensin II, a highly potent vasoconstrictor.
Angiotensin II binding to the membrane-bound GPCR, angiotensin II receptor 1 (AT1), causes vasoconstriction directly via the potentiation of noradrenaline release from sympathetic nerve terminals in blood vessel walls. The downstream consequences of AT1 receptor activation are partially counterbalanced through the activation of AT2 receptors. As a result of the robust vasoconstrictor features of AT1 receptor activation, drugs that function as antagonists at this receptor, including losartan and valsartan, are effectual antihypertensives due to their indirect vasodilator activity.
The inhibition of the angiotensin-converting enzyme (ACE) inhibits the generation of angiotensin II and thus displays antihypertensive effects. Angiotensin II also functions indirectly by triggering the secretion of vasopressin (AVP) from the pituitary gland and augmenting the release of aldosterone from the adrenal cortex. Both aldosterone and AVP increase water reabsorption in the kidney, thus augmenting blood volume and, as a result, blood pressure.
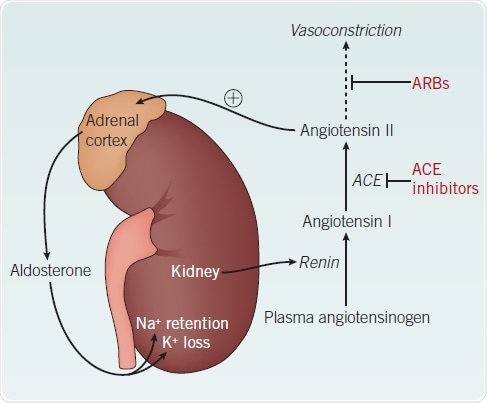
Figure 1. The renin-angiotensin-aldosterone system.
Activation of the RAAS in response to a fall in blood pressure stimulates the release of renin from the kidney. This leads to the production of the potent vasoconstrictor, angiotensin II. Angiotensin II also induces aldosterone release from the adrenal cortex, triggering increased water reabsorption. Together these mechanisms counter the decrease in blood pressure.
Abbreviations: ACE – angiotensin-converting enzyme; ARB – angiotensin II receptor blocker
Aldosterone Receptor
Aldosterone receptor antagonists comprising eplerenone and spironolactone also lead to antihypertensive effects as a result of their inhibitory actions on water reabsorption. The consequent lowering in circulating blood volume thereby reduces blood pressure. Additional diuretics, comprising bumetanide and furosemide, also reduce circulating blood volume and thus blood pressure, although they operate through the inhibition of the Na+/K+/2Cl- cotransporter (NKCC). The NKCC is a key membrane pump that drives magnesium ion and calcium ion reabsorption in the renal medulla, leading to water reabsorption from the loop of Henlé.
In addition to indirect control of vascular tone by RAAS and the sympathetic nervous system, direct control mechanisms in the blood vessel wall also represent a reasonable therapeutic target in hypertension. Endothelin 1 (ET-1), nitric oxide (NO) and prostacyclin (PGI2) are important regulators of blood pressure within the vasculature (Figure 2). Additional major vasodilators, such as bradykinin and acetylcholine, also directly affect vascular tone by instigating the generation of endothelial nitric oxide.
Endothelin Receptors
Endothelin receptors are divisible into two categories – endothelin A (ETA) receptors and endothelin B (ETB) receptors. ETA receptors are absent in the endothelium, while ETB receptors are highly expressed therein, although both receptor subtypes occur on the underlying vascular smooth muscle cells. Activation of ETA receptors by ET-1 causes vasoconstriction while the effects of ETB receptor activation are cell type-specific; smooth muscle cell ETB receptor (ETB2) activation leads to vasoconstriction, yet endothelial cell ETB receptor (ETB1) activation causes vasodilation via the generation of NO and PGI2.
Additional Therapeutic Target in Hypertension
NO is an additional therapeutic target in hypertension, as its bioavailability is regularly impaired among hypertensive patients, which is an indicator of endothelial dysfunction. NO is an essential endogenous vasodilator which is secreted in response to endothelial membrane receptor stimulation by agonists comprising 5-HT, acetylcholine and bradykinin, in addition to shear stress. The activation of endothelial cell membrane receptors by agonist stimulation or shear stress engenders a rise in intracellular calcium ion concentration. This augmented calcium ion availability causes activation of calmodulin (CaM), a calcium-binding protein. The Ca2+-calmodulin complex is an essential factor in the removal of the caveolin-mediated inhibition of endothelial nitric oxide synthase (eNOS), which in turn enables eNOS enzyme activity.
The main reaction of eNOS is the conversion of L-arginine to L-citrulline, which generates nitric oxide as a by-product. NO generation and release from endothelial cells prompt an increase in cyclic GMP concentration in the underlying smooth muscle cells via the activation of soluble guanylyl cyclase (sGC). This, in turn, reduces the intracellular calcium ion concentration, engendering smooth muscle cell relaxation and vasodilation. As a result of the central participation of calcium ions in the regulation of vascular tone, drugs that inhibit the influx of calcium ions through calcium channels following cellular depolarization also directly engender vasodilation. Diltiazem and nifedipine represent typically utilized calcium channel blockers for the treatment of hypertension.
Vasodilators
Vasodilation is also prompted by inhibiting the sensitivity to calcium ions of downstream mediators implicated in vasodilation, such as the Rho-associated protein kinase p160ROCK. Y-27632 is an example of a compound that generates vasodilation through this alternative mechanism. There are additional vasodilators that are secreted by endothelial cells, and which lower intracellular calcium within underlying smooth muscle cells. These comprise C-natriuretic peptide (CNP), through its actions on natriuretic peptide receptors (NPR), and prostacyclin, which operates on I prostanoid (IP) receptors.
Compounds targeting these receptors directly regulate the vasodilatory features of such endogenous mediators. The activity of these endogenous vasodilators can also be adjusted by targeting second messenger signaling molecules, specifically cAMP and cGMP. The β adrenergic receptor agonist formoterol is one example of a compound that increases cytoplasmic cAMP levels, while cGMP levels can be therapeutically augmented by the activation of sGC, utilizing compounds including A 350619 and BAY 41-2272.
Vasodilation is also accomplished via the selective increase of the membrane permeability to K+ ions, inducing cellular hyperpolarization, and subsequent relaxation. Activators of ATP-sensitive potassium channels (Kir6; KATP), including nicorandil and cromakalim, manifest powerful vasodilator activity as a consequence of this mechanism, although the NO donor activity of nicorandil in addition to its Kir6 channel activating properties further enhances its potency as a vasodilator.
Conclusion
In spite of the substantial developments in the identification of relevant therapeutic targets implicated in hypertension, the side effect profiles caused by the ubiquitous expression of many of these targets restricts their long term usage. Instead of causing a direct reduction of circulating blood pressure, emerging ideas regarding the treatment of hypertension are tending to focus on the amelioration of endothelial dysfunction – via mechanisms such as augmenting nitric oxide bioavailability – as a way of reducing the target organ damage linked to hypertension and thus improving prognosis.
Since endothelial dysfunction can be activated by free radical-mediated damage, a number of clinical trials examined the impact of vitamin E administration on hypertensive patients. This research indicated that the antioxidant had little or no beneficial effects, although emerging data is reporting an antihypertensive effect of statins, independent of their lipid-lowering activity. Additional possible therapeutic targets in hypertension comprise the prevention of the neutral endopeptidase-mediated proteolysis of natriuretic peptides, as well as increasing L-arginine levels. However, additional research focus is needed to ensure the development of new, effectual treatments for hypertension.
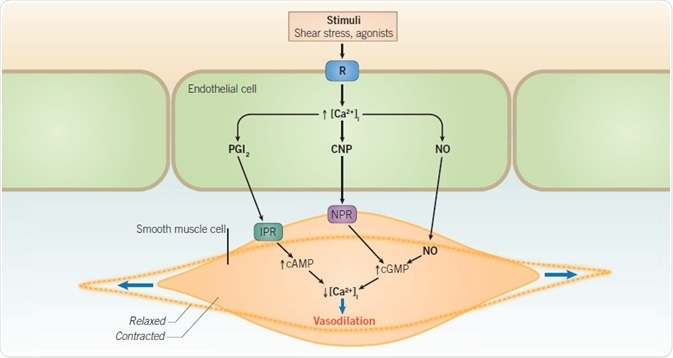
Figure 2. Nitric oxide-mediated vasodilation.
Endothelial cell surface receptor activation by vasodilatory stimuli triggers a rise in intracellular Ca2+ ([Ca2+]i ). Downstream intracellular signaling pathways lead to the generation of vasodilatory mediators including PGI2, CNP, and NO. By binding to their respective receptors on the underlying vascular smooth muscle cell membranes, these mediators prompt a decrease in intracellular calcium within vascular smooth muscle cells through the actions of second messengers including cAMP and cGMP. Decreased intracellular calcium triggers vascular smooth muscle cell relaxation and subsequent vasodilation.
Abbreviations: cAMP – cyclic adenosine monophosphate; cGMP – cyclic guanosine monophosphate; CNP – C natriuretic peptide; IPR – I prostanoid receptor; NO – nitric oxide; NPR – natriuretic peptide receptor; PGI2 – prostacyclin; R – receptor.
About Tocris Bioscience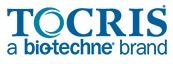
Tocris Bioscience is your trusted supplier of high-performance life science reagents, including receptor agonists & antagonists, enzyme inhibitors, ion channel modulators, fluorescent probes & dyes, and compound libraries. Our catalog consists of over 4,500 research tools, covering over 400 protein targets enabling you to investigate and modulate the activity of numerous signaling pathways and physiological processes.
We have been working with scientists for over 30 years to provide the life science community with research standards, as well as novel and innovative research tools. We understand the need for researchers to trust their research reagents, which is why we are committed to supplying our customers with the highest quality products available, so you can publish with confidence.
Tocris is part of the protein sciences division of Bio-Techne, which also includes the best in class brands R&D Systems, Novus Biologicals, ProteinSimple, and Advanced Cell Diagnostics. Bio-Techne has united these brands to provide researchers with a full portfolio of research reagents, assays, and protein platforms. For more information on Bio-Techne and its brands, please visit bio-techne.com.
Sponsored Content Policy: News-Medical.net publishes articles and related content that may be derived from sources where we have existing commercial relationships, provided such content adds value to the core editorial ethos of News-Medical.Net which is to educate and inform site visitors interested in medical research, science, medical devices, and treatments.