Immunoengineering is a portmanteau of immune engineering and refers to a new discipline that helps to create engineering tools to both investigate and change the immune system. Immunoengineering is considered to be a revolutionary tool in medicines including oncology, autoimmunity, infectious diseases, transplantation, and others.
Immunoengineering is an important tool as dysfunction of the immune system plays an important role in several diseases ranging from inflammation to cancer through to autoimmunity and transplant rejection. Therapies based on immunomodulation and engineering strategies are therefore instrumental in the treatment of these diseases.
Immunoengineering allows the quantitative control of immune cells and their signaling to both strengthen the understanding of the immune system whilst developing new immunotherapies.
Immunoengineering approaches target several different cell types of the immune system including dendritic cells and T cells as they are key mediators of adaptive immunity. Dendritic cells function as sentinels; they continuously migrate through tissues and sample contents for the presence of foreign antigens before migrating to the lymph nodes where they present antigens to T cells.
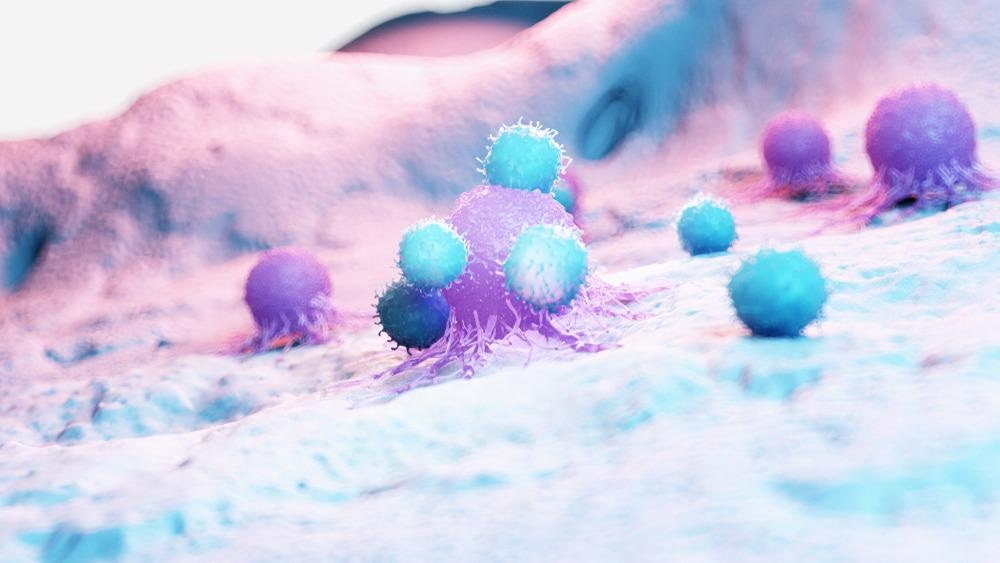
Image Credit: SciePro/Shutterstock.com
Immunoengineering for vaccines and therapeutics
To effectively combat infectious diseases, new vaccines with enhanced safety, efficacy, and scalability are needed. Immunoengineering allows these types of vaccines to be constructed out of a range of diverse materials including liposomes carrying DNA to protect against malaria, and biodegradable polymeric nanoparticles that can act as a nicotine vaccine.
Moreover, immunomodulatory molecules can be engineered to include glycosylation, a type of glycoengineering that can be used to enhance the immunogenicity of antigens. Collectively these help further enhance the immunogenicity of antigens.
Another component of vaccines is the route of administration. Biomaterials can be used to construct a range of microneedles capable of painlessly administering vaccines safely and easily. The use of biomaterials is also expanding vaccine translation, namely developing mRNA vaccines for infectious diseases including SARS-CoV-2.
Emerging areas of research in immunoengineered vaccine includes developments in cancer vaccines. In this approach, the therapy is aimed at a select few target immune cells, where the therapeutic can train the immune system to activate, expand (proliferate), and produce a systemic anti-cancer cellular immune response throughout the body.
An example of this approach is an mRNA vaccine which encodes a tumor antigen that can be intracellularly delivered in nanoparticles. When these nanoparticles reach dendritic cells, these cells can induce cytotoxic t cells to kill triple-negative breast cancer; This has been demonstrated in a mouse model.
Alternatively, vaccines can target the tumor microenvironment, causing it to be reprogrammed in situ using biodegradable nano immunomaterials to generate antigen-specific systemic cellular immune responses. This is beneficial because no prior knowledge OF cancer-specific antigens is needed.
The engineering of immune cells ex vivo (outside of the body) is another example of immunoengineering. This is particularly relevant in chimeric antigen receptor (CAR)-T cell immunotherapy for the treatment of several councils including multiple myeloma.
To further improve performance, immunoengineering approaches use molecular engineering which involves creating immunomaterials to boost the delivery of CAR-T cell activity in vivo and using this genetic encoding to add new functions.
An example of this is the genetic encoding of critical proteins like cytokines or by incorporating cellular imaging modalities so that cells can be tracked in terms of location and phenotype. For imaging, cell labeling can be genetically encoded in and engineered CAR T cells using herpes simplex virus type 1 thymidine kinase. This form of engineering allows visualization by PET imaging on Firefly luciferase.
Immunoengineering for cell regeneration
Immunoengineering approaches allow new pathways for healing to be possible. Regenerative immunology leverages immunoengineering approaches to survey immune cell interactions and design improved biomaterials. An example of this is the use of a synthetic material called polycaprolactone, to increase the quantities of T helper 17 cells, the production of interleukin 17, and the activity of other immune cells including macrophages that create fibrosis. This promotes tissue repair.
Immunoengineering is also used to mimic the effect of the immune system cells, i.e. macrophages. Macrophages promote healing responses and precise macrophage phenotype switching can facilitate this control. Using biomaterials-based research, localized donor-specific immune tolerance in allotransplantation can be achieved using microparticles that release a molecule called CCL22 to recruit T cells.
Immunoengineering can also be used to prevent transplant rejection as well as the rejection of implanted devices. Synthetic biomaterials coat the surface of the implant, and through surface conjugation which prevent foreign body responses to implanted devices. For example, researchers have created a Fas-ligand immunoengineered biomaterial that causes apoptosis of T effector cells and induces localized immunomodulation to facilitate graft survival of islet in diabetic mice.
The history and future of immunongineering
The immunongineering field is relatively new, with the earliest use of the term tracing back to 2012, where it featured in a science translational medicine article that described “the nascent field of immunoengineering aims to provide new approaches to understanding, application, and therapeutic manipulation of immunology.”
Before the article in 2012, the terms immunongineering and immune engineering were rarely found in the literature. Since 2012, it is become increasingly used in scientific literature, with the Society For Biomaterials (SFB) forming a Special Interest Group (SIG), “Immune Engineering,” in 2015. This was founded in response to the long-standing interests of engineers and scientists in biomaterial research across interests and disciplines as tissue engineering, drug delivery, and implantable devices.
The purpose of the Immune Engineering SIG is to provide a hub for professional interaction for academics and industrial research groups. As such, immunoengineering scientific events have been hosted that have allowed the discipline to grow.
Limitations, challenges, and opportunities of immunoengineering
The field of immunoengineering is new, having only produced published research over 9 years, and as such presents, several opportunities as well as being faced with challenges.
The most persistent challenge in the field is the limitation of current animal models; some models that might work particularly well when examining a therapeutic response may not be relevant to a human patient with the same disease as a result of several factors such as the use of immunocompromised animals, differences in physiological metabolism and transport, and differences in the tumor microenvironment.
As such future experimentation offers the opportunity to expand into in vivo animal experiments with engineered humanized immune systems, to ex vivo systems of tissue-engineered human-like organs-on-a-chip. Moreover, in silico multi-scale and multi-physics models offer the opportunity to stimulate aspects of patient characteristics. Collectively, these new engineering technologies can lead the way in immunoengineering research.
The immune system is also complex because of the involvement of many organ systems and individual cells that govern specific immune responses. For example, macrophages encompass a wide range of activities ranging from the stimulation of inflammation and neutralizing pathogens to wound healing and tissue repair as well as anti-inflammatory activity and immune regulation.
Other areas of research further add to the complexity because as they increase the repertoire of cellular function. For example, the engineering CARs on the surface of macrophages to expand their cellular function for cancer immunotherapy. Despite these challenges, this also offers the opportunity to understand the mechanisms and function of the immune system. Specifically, engineered technologies could be used to characterize individual immune cells via multiplexed imaging and RNA sequencing combined with computational tools.
Over the past nine years, there has been a rapid growth in immunoengineering. It brings together several disciplines including biomedical engineering, immunology, medicine, biology, chemistry, material science, computer science, and chemical engineering to create a new discipline. Dedicated immunoengineering centers in educational institutes are becoming increasingly common, with increased grant funding and investment to produce high-impact research and transnational biotechnologies for development.
The field promises to alter existing the way diseases on treated – from cancer to infectious disease – while also enabling new approaches for treating others, such as diabetes, multiple sclerosis, and autoimmune disease. As new tools are discovered, and a greater understanding of the function of the immune system is obtained, the field also promises to improve transplantation protocols as well as the quality of medical devices. Despite these promises, there are several complex challenges to overcome that require multidisciplinary collaboration to enable immunoengineering to continue to grow and reach its full potential.
References:
- Swartz MA, Hirosue S, Hubbell JA. (2012) Engineering approaches to immunotherapy. Sci Transl Med. doi:10.1126/scitranslmed.3003763.
- Niederer SA, Lumens J, Trayanova NA. (2019) Computational models in cardiology. Nat Rev Cardiol.. doi:10.1038/s41569-018-0104-y.
- Fisher JD, Zhang W, Balmert SC, et al. (2020) In situ recruitment of regulatory T cells promotes donor-specific tolerance in vascularized composite allotransplantation. Sci Adv. doi:10.1126/sciadv.aax8429.
- O'Brien EM, Risser GE, Spiller KL. (2019) Sequential drug delivery to modulate macrophage behavior and enhance implant integration. Adv Drug Deliv Rev. doi:10.1016/j.addr.2019.05.005.
- Buettner MJ, Shah SR, Saeui CT, et al. (2018) Improving Immunotherapy Through Glycodesign. Front Immunol. doi:10.3389/fimmu.2018.02485.
- Allen TM, Brehm MA, Bridges S, et al. (2019) Humanized immune system mouse models: progress, challenges and opportunities. Nat Immunol. doi:10.1038/s41590-019-0416-z.
Further Reading