Photoacoustic tomography provides information about tissues in real time by utilizing ultrasonic waves. A contrast agent is used which interacts with light, creating ultrasonic waves. One such contrast agent is gold nanoparticles.
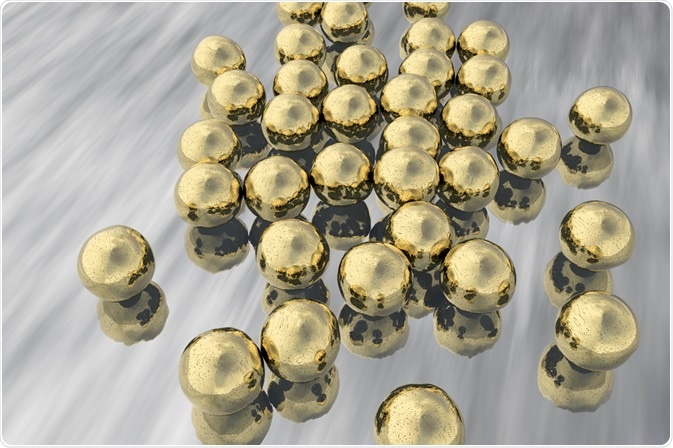
Kateryna Kon | Shutterstock
Photoacoustic tomography is different to ultrasound imaging, which uses sound waves emitted and detected by a device outside the body and relies on the properties of biological tissues to generate an image.
This type of imaging offers greater resolution and deeper imaging than fluorescence techniques while minimizing the disadvantages of methods like positron emission tomography which expose the patient to dangerous radiation.
How does photoacoustic tomography work?
When a laser strikes an object, the object rapidly heats and expands, producing an acoustic wave. A transducer is capable of detecting these waves and processing them to form an image electronically.
Certain wavelengths of light in the near infra-red region are able to penetrate through biological tissue, with minimal diffusion and scattering to depths of up to five or six centimeters at laser intensities that are incapable of damaging human skin.
The force of these waves are picked up by the transducer depending on the extinction coefficient of the material on which the converging laser beam is focussed.
The laser can be focussed on more shallow biological features, such as skin depth capillaries, with excellent resolution, or sacrifice some of that resolution in order to image more deeply into the body.
Using gold nanoparticles as contrast agents
Gold nanoparticles are plasmonic species, meaning that an electric field of incident light is able to induce collective oscillations of the gold nanoparticle’s electrons, leading to a phenomenon known as localized surface plasmon resonance.
This phenomenon greatly enhances the extinction cross section of the particle, meaning that more light is able to interact with it. Two main interactions with a gold nanoparticle are possible for incoming photons: scattering or absorption.
When light is scattered, it radiates away from the gold nanoparticle; otherwise, it is taken in and usually becomes heat. Light waves that are in-phase with the oscillations of electrons around the gold nanoparticle are absorbed, while others are scattered.
Spherical gold nanoparticles of around 5-30 nm possess a surface plasmon resonance wavelength of approximately 520 nm, getting larger as the diameter of the particle is increased. As the distance between the negative electron cloud-positive metal particle dipole points is increased then the frequency of oscillation also lowers, meaning a lower frequency and higher wavelength of light is in phase with these oscillations and thus absorbed by the gold nanoparticle.
The shape of a gold nanoparticle can be tuned further to push this absorbed wavelength into the ‘tissue transparency window’ by the creation of rod, star, cubic, hollow, cage, or prism geometries, among others.
This allows them to be optimally detected at depth using photoacoustic tomography since laser light of this wavelength will be absorbed by the particle, which will go on to heat and expand. These tiny expansions and contractions generate ultrasonic waves, allowing a detailed picture to be created.
Gold nanoparticles are non-toxic and can be coated with ligands, allowing them to actively target a tumor. This is in addition to the enhanced permeability and retention effect demonstrated by tumors that leads to the accumulation of gold nanoparticles within them, for greater lengths of time than small molecule contrast agents due to their larger size.
Further Reading