An interview with Assoc. Prof. James Rabeau and Dr. Louise Brown conducted by James Ives (MPsych)
Can you give an overview of LuciGem’s technology for the illumination and tracking of small molecules using fluorescent diamonds and phosphorescent nanorubies?
Many of the most fundamental, life governing biological processes take place at size and time scales that remain invisible with current imaging technologies. Understanding how the molecules that underlie these processes move, interact and change could pave the way for new treatments, cures and the development of novel drug therapies.
This can only be achieved by seeing these molecules in action in their own environments. Currently one of the main shortcomings of other probes available in biotechnology is often their inability to perform in living environments.
At LuciGem we use small and bright nanodiamond and nanoruby probes which can be attached to objects (molecules and cells) to light them up and thus enable tracking using optical or other detection techniques over long periods.
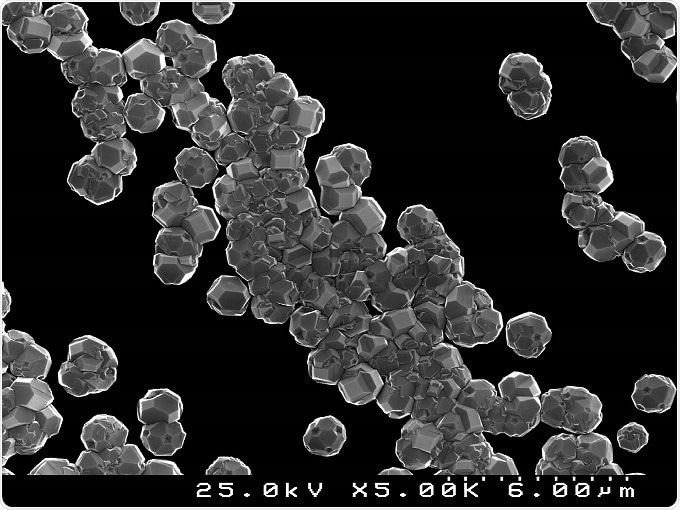
Nano diamond particles, grown synthetically in the lab. The crystals can be seen clearly, on an average being about 100nm in size. CC 4.0 D. Mukherjee.
A good analogy is the way mobile telephones can be used to track your location using GPS. In conjunction with other key technologies, this type of probe will help to unravel new depths of knowledge associated with complex biological processes.
Why were these nanomaterials chosen? What properties do they hold that make them suitable for biomedical and life science applications?
The unique value of nanodiamonds and nanorubies, in the context of imaging and tracking, is that they exhibit perfect brightness, have superior biocompatibility and have ‘tunable’ surface structures that can be modified to target most molecules of interest.
As a result, the applications for the life-science researcher, across the fields of chemistry, physics, biology and medicine, using such novel materials are endless.
Furthermore, with the diamond lattice primarily comprised of carbon atoms, these materials are as close to biocompatible as can be, making them well suited to biological and biomedical applications.
The presence of “color centers” and impurities in the diamond lattice means they can be readily detected using fluorescence microscopy.
An example of one such color center that can be found in a nanodiamond crystal is the nitrogen-vacancy (NV). This single imperfection is created in the lattice of the diamond crystal which traps an unbound electron and gives rise to a well-defined electron spin polarization state.
What is the research history of these nanoparticles? How has this research progressed from Macquarie University into commercial applications?
Diamonds have always been of interest because of scarcity and extreme mechanical properties like hardness and thermal conductivity. However, it was not until the 1950’s that commercial applications for diamonds really took a turn when researchers at the General Electric Company worked out how to make them in a lab.
Today, nanodiamonds are produced using a wide range of techniques including detonation synthesis, plasma-assisted chemical vapor deposition and high-pressure high-temperature synthesis, to name a few.
However, while most of these approaches can supply nanodiamonds in large quantities for industrial applications, obtaining diamond material for life-science applications is still challenging.
Groups around the globe have made considerable advances in the preparation of nanodiamonds and there are an increasing number of commercial organizations becoming established in the space.
At Macquarie University, we have developed a methodology that now allows us to reproducibly isolate and process nanodiamonds of desired sizes and surface properties for a range of sizes below 1 micrometer, to as small as ~5nm.
Our clean nanodiamond material, which contains color centers such as the NV center, is currently being used for quantum technology applications, optical trapping, fluorescence resonance energy transfer and biological imaging.
However, it is the ability to continually image and track a targeted biomolecule of interest that is most exciting, not only for basic sciences, but for the development of improved diagnosis and therapeutic approaches.
What is currently the gold standard in imaging techniques to view and image the smallest particles?
Organic based probes are still the most extensively used class of fluorescent biological makers for imaging purposes. These sub-nanometer sized fluorescent probes create high optical contrast in biological samples where other techniques such as bright-field or phase contrast microscopy fail.
However, they fail in that they readily photo-bleach which limits data and imaging collection to only a few seconds, at most. Other engineered fluorescent materials are available that can provide superior photostability, such as semiconductor nanocrystals, also known as quantum dots.
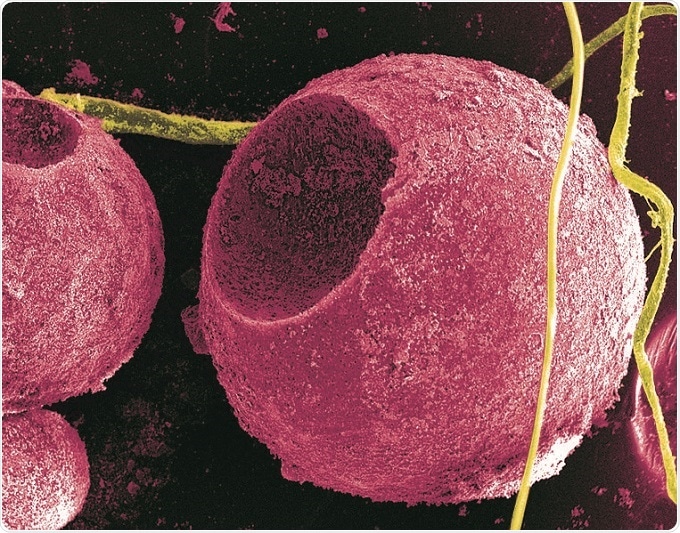
Cadmium sulfide quantum dots on cells.
While quantum dots have been in the market since the early 80’s, their composition of toxic elements has typically prevented them from use for in vivo applications. They are still predominantly used in research labs.
Other fluorescent nanoparticles include doped silicas, hydrophilic and hydrophobic organic polymers, carbon dots, upconversion nanoparticles, and noble metal nanoparticles such as nanogold and nanosilver.
Choosing the ideal probe from this group always depends on the application and specific criteria.
What are the limitations in seeing biological processes on a small scale?
The ability to see on the small scale, down to the level of single molecules, was notably recognized by the awarding of the 2014 Nobel Prize in Chemistry to Stefan W. Hell, Eric Betzig and William E. Moerner.
This trio developed two different techniques for increasing the power of light microscopy, allowing scientists to see molecules in action within a living cell, and therefore overcoming the “diffraction limit” which meant that details within a cell stopped being seen at approximately half the wavelength of visible light (~0.2 µm).
While we can see smaller than this, using electron microscopy, this can only be achieved on non-living samples. However, cells, and the biomolecules in them, are dynamic entities. Tracking and following such dynamic biological processes in real time is the current limitation.
Compared to other bioimaging probes, what does LuciGem’s product suite offer to improve imaging?
For quantitative long term imaging, nanodiamonds and nanorubies are excellent materials. The fluorescent signal from nanodiamond and nanoruby never expires, meaning detection can be carried out over extended periods that are not likely accessible using other widely available probes.
This property, and the non-toxic nature of our materials produced at LuciGem, enables 4D imaging in living systems. As shown by a recent study, our materials are also the brightest emitters on the market by almost two orders of magnitude.
Please describe the therapeutic and biomedical applications?
By modifying the nanodiamond and nanoruby surfaces and functionalities by attaching various biomolecules of interest for interaction with the targets, these probes can be used to not only image regions of interest in vivo but can also be used to deliver drugs where required in the body.
Interestingly, the spin resonance signal of the trapped electron in the NV color center is also temperature dependent, opening up new possibilities to use these probes as nanometer-scale thermometers in a living cell.
Efforts to use this material where long-term cellular fate mapping is critical, such as stem cells, are also being explored. As a non-toxic platform, the possibility as imaging or drug delivery agents appears to be endless.
What does the future hold for LuciGem and new nanomaterial production?
Right now, we are making nanoparticle probes that allow biotechnology companies to track drugs and therapeutics in living systems. These particles are non-toxic and can be detected by fluorescence continuously.
Our vision is to help people live longer and healthier lives by making an impact in life-sciences and new therapeutic products. We have a solid scientific base and understanding of some pretty unique and advantageous materials that are starting to gain a foothold in real-life applications. Along with many others around the globe, LuciGem is pioneering some of these applications.
About Assoc. Prof. James Rabeau
James is a physicist with a unique combination of research and business expertise having worked in both academia and commercial organizations.
He has led research teams in quantum and biological applications and fabrication of nanodiamond materials.
About Dr. Louise Brown
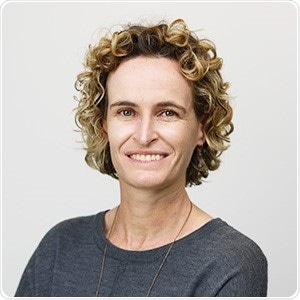
Louise is a physical chemist with extensive experience in the field of biolabeling. She is passionate about developing new tools for bio-imaging to better reveal dynamic processes within cells and other living systems.
Since 2007, James and Louise, together with post-docs and students, have pioneered the development of diamonds for biological imaging.