Sponsored Content by PromoCellReviewed by Alex SmithMar 22 2022
In this interview, News-Medical talks to Dr. Glauco Souza and Linda Boekestijn about their work in the wound healing space and how they devised a new high throughput 3D model for wound healing.
Can you provide a brief overview of your background and your work in wound healing?
Glauco Souza: My position is Director of Global Business Development & Innovation, 3D Cell Culture for Greiner Bio-One. I was one of the inventors of magnetic 3D cell culture by levitation and bioprinting – the primary techniques we perform in wound-healing assays.
Linda Boekestijn: I did an internship for my Master’s degree in Biomedical Engineering at CytoSMART Technologies from May 2021. I studied Biomedical Engineering in Eindhoven, before graduating in August 2021.
I started working at CytoSMART Technologies as an Application Scientist in October 2021. My work focuses on helping existing or potential customers evaluate their research, see which devices we have, and what opportunities we can provide for them.
I also test the devices via different types of in vitro experiments, exploring opportunities to connect with other companies like Greiner Bio-One. I’m new to the field, but I already really like it.
What sort of biological healing mechanisms do a wound-healing model target?
Glauco Souza: People use the term ‘wound-healing’ a little bit loosely. I am chemist working in Bioengineering for the last 15 years. The wounding-healing assay is an assay that mimics what wound healing looks like, but it is used more broadly.
The assay we have developed is a circle with a hole in the middle, and over time, this hole is closed, much like a wound would close. When people think of a wound, they generally think of epithelial cells, fibroblasts and the other cell types that close a wound.
But we could use a single cell type – for example, muscle cells – and still call this a wound-healing assay. The concept of wound healing comes from the closure of the empty space, and by imaging this process, we can get useful quantitative information.
Linda Boekestijn: Wound healing models, in general, target the wound healing process. For example, when you have a scratch, you get healing of the skin. When everything goes well, you form new skin, and everything is organized properly. However, often, this is not happening, and people are left with scar tissue or chronic wounds.
Wound healing models are used to investigate potential drugs to help wound healing with the formation of scar tissue or chronic wounds. We target the four-phase wound healing process that is performed by a healthy body: hemostasis, the inflammatory phase, migration or the proliferative phase, and the maturation phase.
We try to target all four phases in the wound healing process, but generally, in vitro research will focus on one of these specific phases, or it can be unclear which phase the research targets.
What are some limitations of existing 2D in vitro wound-healing models?
Glauco Souza: As I mentioned, people use the term ‘wound healing’ very loosely. This is especially the case with the 2D approach. The 2D approach involves growing cells on a monolayer - cells are placed in a plastic container and grown flat on the bottom.
This technique is also known as the ‘scratch assay,’ where researchers scratch the surface and create a gap in the culture. In time, this gap is filled by cells growing into it. Here again, cells fill up a space, referring to this as wound healing.
The problem with this process is that it is not true wound healing as you are just looking at cells migrating and growing on plastic. There is nothing especially biological going on here, and it is very hard to simulate complex wound-healing processes which involve different cell types using this method.
For example, if you mix fibroblasts with other cell types in a monolayer, fibroblasts will take over the culture, causing population loss.
Often, you’re just looking at the cells interacting with plastic, which does not offer much value compared to other models that simulate wound-healing from a truly biological perspective.
Linda Boekestijn: While 2D in vitro models are often used in many settings, a significant disadvantage of these 2D models is that they can’t mimic the native cellular environment. The human body is 3D and cells are in contact with each other and the surrounding tissue. It is widely known that 2D models cannot mimic the 3D environment that we have in the body.
The outcome using a 2D assay can be different from how a drug would behave in your body. When used in a Drug Development Process, this can lead to false outcomes in the later phases of the development of these drugs because you have used these 2D models.
The main limitation of 2D models is that they cannot mimic the native cellular environment, meaning that these models miss the cell-cell interaction and cell-matrix interactions.
What are some advantages that a 3D wound-healing model provides biomedical researchers?
Glauco Souza: 3D is a more natural approach. We are 3D, so there is cell-to-cell interaction in three-dimensional space in vivo. We want to model in vitro biology that best replicates how in vivo biology works in reality.
We know that cell-to-cell interaction between cells of the same type and different types defines the biology of the tissue, but on a 2D monolayer culture, it is not easy to see this 3D aspect of the biology.
The valuable part of doing this in 3D is that we can also bring in different cell types that can interact, allowing us to have a more realistic model that better reflects in vivo biology. The goal is to develop a more predictive model in 3D, taking into account all the available data.
Linda Boekestijn: 3D models are better at mimicking the native cell environment, and we can do this in different ways. We can make scaffolds, use hydrogels or use the technique that we did - the bioprinting technique.
With these 3D models, the cells can be placed inside a 3D environment or the cells can form their own extracellular matrix (ECM) products like collagen. Also, cells can interact with other cell types which mimics the native cell environment.
What factors make it challenging to set up a 3D model?
Glauco Souza: There are different challenges with 2D modeling and 3D modeling. When working with 2D biology, you work with cells that adhere to plastic, making it very easy to change media, move media or downstream applications like immunohistochemistry or immunofluorescence.
When you move to 3D modeling, you lose that ease of transportation. Changing media is more complex. People can use gels, but gels present a range of complications that make manipulation more difficult.
That is where magnetic 3D cell culture comes in. We use magnetic fields as a surrogate for cell attachment in 2D. We decorate the cells with magnetic nanoparticles – we call this the NanoShuttle – and then we use a ring magnet to guide the cells into the shape of a ring with a hole inside – a toroid type of geometry.
We can then observe the cells closing as a three-dimension structure. The magnetic field enables us to manipulate the cells, position these and create a shape that we can follow.
Linda Boekestijn: There are a lot of opportunities with the 3D models, but setting up these models requires a lot of time. When using scaffolds or hydrogel, we need to exclude many factors that can influence the cell so that we can ensure that we know what causes the change in cell behavior.
This requires a lot of testing, time, as well as some lab experience and experimental skills. Therefore, we were very enthusiastic to use the bioprinting technique from Greiner, this technique is relatively easy and fast compared to other 3D development techniques.
How do magnetic 3D bioprinting techniques compare to 3D scaffolds?
Glauco Souza: It is very hard to create a pattern when putting cells on a scaffold. You can see the cells migrating, but it is challenging to generate a 3D structure, populate the cells precisely, and leave space for the cells to grow or close in to mimic a wound healing process.
The magnetic field I mentioned allows us to create a precise shape externally without going to the well, and that will enable us to monitor an image in real-time and get a lot of information from this process.
Can you briefly describe the combination of systems that were used to develop a 3D in vitro wound-healing model in this study?
Glauco Souza: In this application, we used the CytoSMART System to get real-time information.
High throughput is also important, so we used a 384 well system with small ring magnets under each well to generate these wound-like structures. Cells can rapidly regenerate these ring patterns, and we can image these over time to get quantitative data regarding the wound culture.
We also took this a step further by adding different cell types, allowing us to clearly see the difference between configurations based on different cell types and cell ratios, suggesting that wound closure behaviors varied greatly.
This represents another enabling tool for us to tune the composition of the culture, allowing us to achieve a more predictive result.
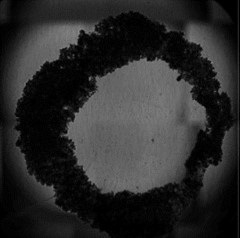
Fig. 1: (Left) A 96-well plate placed on the CytoSMART® Omni inside a humified incubator. (Right) 3D ring construct representing a dermal wound.
Linda Boekestijn: To develop this high-throughput 3D wound-healing model, we needed to magnetically label the cells.
We used all of the techniques and the protocols from Greiner Bio-One to label and levitate our cells. This ensured that the cells were active, and they started to create extracellular matrix products (for example, collagen or glycosaminoglycans) representative of native cellular environments.
After that, we resuspended our cells in a 384-well plate and placed a plate from Greiner Bio-One underneath it. That plate had 384 magnetic rings on it, ensuring that our cells formed these 3D ring constructs.
We added different growth factors to test what the effect was. The rings comprised different ratios of the co-culture of two different cell types to create or mimic the different phases in the wound healing process, and then we put this plate on the CytoSMART Omni.
We placed the CytoSMART Omni inside the incubator, allowing us to scan the complete well plate over time. All wells were scanned inside the incubator, without affecting the natural cell environment.
When the Omni scans the well plate, it creates many images that are automatically uploaded to our CytoSMART cloud. In the cloud, we used the algorithm to automatically measure the internal ring area.
As the ring closed, we were able to automatically measure the internal ring area to see the closure speed over time.
What are some of the advantages of using PromoCell keratinocytes and fibroblasts?
Linda Boekestijn: When we initially started to talk with PromoCell, they were accommodating, and they also shared a lot of experience with cells, cell media, and what we needed to use for this task.
This was important because we used a co-culture of keratinocytes and fibroblasts, and we needed to decide which medium to use because they both typically grow in different culture mediums. Their advice helped us achieve optimal culture conditions for the cells.
What are some advantages of CytoSMART’s live cell imaging device?
Linda Boekestijn: As the device is placed inside the incubator during imaging, the cell culture is not affected by anything other than the treatments you are giving because it stays in its optimal cellular environment.
You can choose your preferred time interval, and we do live cell imaging which provides more information than an endpoint assay.
The Omni automatically scans at those defined time points, acquiring a couple of thousand images. These images are uploaded to the CytoSMART cloud, where they are stitched together, ensuring that we have a high resolution.
This is especially important when working with the 3D wound-healing models because we need to see where our cells are, ensuring these are attached to the 3D constructs and that everything is nicely aligned.
As images are stored in the cloud, there is no need to go to the lab to check results - you can view them from your laptop or phone and see how they are doing, only going to the lab when something needs to be changed.
Also, because we use this automatic tool to measure the internal ring area, this saves time and results in a highly automated workflow.
Finally, the Omni can do full-well imaging, meaning that we can see full wells, this makes sures that your 3D structures cannot move out of your field of view.
Why is investigating different phases and cell types in wound healing important?
Linda Boekestijn: As I mentioned, the wound healing process comprises four different phases, each with different functions. Drugs designed to assist one healing phase may have a negative effect on another phase, so it is important to investigate all the different phases as part of drug development research.
It is also important to know in which phase your drug is beneficial because then it can be given to a patient presenting problems in that phase.
Keratinocytes and fibroblasts can stimulate each other in proliferation and migration, so we need to investigate the interaction between those cell types to get a good idea of what the potential drug would do during this process.

The presence of keratinocytes, fibroblasts, glycosaminoglycans (GAG) and collagen in all phases of the native wound healing process. Image credit: Promocell GmbH
How can this 3D wound-healing model be applied in a clinical or biomedical research setting?
Glauco Souza: Applications for wound-healing models and 3D cell cultures continue to be developed for clinical and biomedical research settings. We have worked with collaborators looking at biochemically characterizing 3D cell cultures to see how this resembles actual wound-healing processes.
Our simulated wounds are made the same, literally and figuratively – for example, wounds from diabetes, burning and car accidents.
First, we have to determine that we can recreate biology in a predictive way, and that is what we are currently focused on while also looking to adapt to more clinical settings such as drug discovery and personalized medicine.
We have also used different cell types, like fibroblast and epithelial cells, for vasoactivity assays, looking at drug interaction with vascular muscles.
This work is essential because the primary assay to look at the drugs’ effects on vasoactivity is the aortic ring assay.
In this assay, we take the aorta from an animal, chop it up into rings, and stretch these to see if the drug is a vasodilator or a vasoconstrictor. It is not high-throughput, and there is a lot of variability in animals, so animal cells are not good predictors of human biology.
However, we can use human cells to create these rings and look at vasoactivity. We call this bioassay the “O” ring because it is not just a wound-healing assay; it can also be used in different configurations to look at cell-to-cell interaction, cell mobility and cell migration.
Linda Boekestijn: This method may be helpful in drug development for new wound healing therapies, specifically in the early stages of this process before the clinical stage or in vitro animal testing.
Using 2D in vitro models in the first phase leads to a lot of false outcomes in later phases. We hope that with this 3D model, the number of false outcomes will decrease. This will save time, effort and money in the subsequent phases of research because we can determine which drugs are not a good fit for an application in the early stages.
What would you need to employ this application in a high-throughput process? Or can you already use it in a high-throughput process?
Glauco Souza: We are using a high-throughput 384 well process now. Increasing this to 1,536 wells would be challenging because the magnets would be very small, but 384 is plenty. It is already a much higher throughput than other tools for wound healing.
The imaging system must also be fast enough to collect image data in a high-throughput manner. The CytoSMART approach meets both of these considerations. As such, it is proving to be a valuable tool for high-throughput screening.
What would you want readers to take away from this study?
Glauco Souza: People often forget that time is very important in biology. Seeing how cultures are moving, changing shape and how cells are interacting in real-time is very valuable yet underexplored. We have introduced a tool that enables us to do difficult things in much simpler ways by simply using magnetic nanoparticles on the cells.
Linda Boekestijn: I think the readers should be amazed by the fact that we created a high-throughput 3D model that is relatively easy and fast and that we are performing all of this inside the incubator without affecting our cells too much.
We wanted to show the importance and advantage of using live-cell imaging. We used different ratios of the co-culture of keratinocytes and fibroblasts to mimic the different phases of the wound healing process.
We saw that different ratios lead to different degrees and speeds of wound closure, and the way they migrated and contracted was very different. These differences could only be seen using live-cell imaging.
About Dr. Glauco Souza
Dr. Glauco R. Souza is the Director of Global Business Development and Innovation, 3D Cell Culture at Greiner Bio-One and former President and co-founder of Nano3D Biosciences, Inc. (n3D). He is a co-inventor and inventor of eleven magnetic 3D cell culture patents, including magnetic 3D bioprinting and levitation technologies. Now, Glauco’s mission is to advance magnetic 3D cell culture into a routine laboratory tool that will measurably improve the drug discovery process, cancer research, personalized medicine, and regenerative medicine.

About Linda Boekestijn:
Linda Boekestijn started as an application scientist at CytoSMART in October 2021. Linda studied Biomedical Engineering at the Technical University of Eindhoven, during the last part of her master's she did her externship at CytoSMART. In this period, she developed a new high-throughput 3D wound healing model.
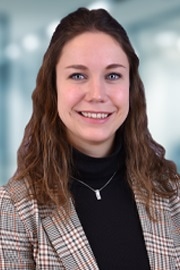
About PromoCell
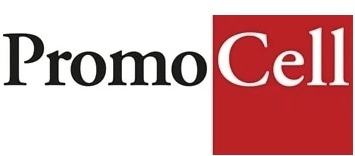
At PromoCell, we help scientists do better research with a world-class portfolio of human primary, stem and blood cells as well as optimized cell culture media. With over 30 years of expertise, we are recognized globally for supplying scientists with the tools and support they need to do groundbreaking research.
All our products comply with European biomedical conventions, ensuring human rights and donor privacy are always protected. Our ISO certifications demonstrate our absolute commitment to quality and our EXCiPACT™ GMP certification enables us to produce our cell culture media and reagents according to GMP standards as a manufacturer of pharmaceutical excipients.
Each year 600 peer-reviewed publications feature PromoCell products. We operate in over 40 countries around the world, helping scientists with all of their research needs.
Learn more about PromoCell on our website, or connect with us on Facebook, YouTube, Twitter or LinkedIn.