Non-healing wounds continue to cause significant patient burden. This article outlines a new 3D wound healing model that is committed to enabling the evolution of novel treatments to encourage wound healing.
The skin usually receives little attention, yet it is a highly advanced organ. It is rich in immune cells to defend against opportunistic microbes and forms a crucial barrier between the body and its environment.1,2
It also allows humans to adjust to environmental changes such as temperature regulation and connect with their surroundings through touch.3,4 The skin is crucial for survival, and this is made clear through its capacity to regenerate.5
Medical intervention is required in cases where skin damage is too significant for it to regenerate, for example, through major surgical excision, burns and trauma, or where local skin conditions continually inhibit effective healing such as ulceration.
This medical intervention can take the form of maggots, negative pressure wound therapy, bioengineered skin scaffolds or growth factors.6,7,8
Chronic wounds continue to be a significant challenge despite the various treatments that are available for assisted wound healing.9
Current treatment measures for severe or chronic wounds are broadly expensive and painful, along with not always being able to achieve effective wound healing.
Enhanced knowledge of the clinical and biological processes that support wound repair is required to advise the development of new effective treatment techniques for non-healing wounds.
Skin wound models that precisely mimic in vivo healing mechanisms and real skin tissue are required for this type of research.
Models for studying wound healing
A complex sequence of events is involved in wound healing that starts with hemostasis which provides a scaffold for new skin growth and stems the bleeding.
This instigates the inflammatory phase, which includes monocyte transformation and neutrophil recruitment, leading to the central proliferative healing mechanism, during which the vascular network is restored, and granulation tissue is created.
Lastly, in the maturation stage, local fibroblasts enter the area, which initiates re-epithelialization.
To understand how best to support and encourage healing, the interactions between different types of cells must be characterized, particularly keratinocytes and fibroblasts, that arise during each stage for complete and correct wound healing and closure.
To enable the research required to gather this knowledge and to evaluate possible new treatments in a cost-effective and ethical manner requires advanced skin models that come close to reproducing in vivo conditions.
Challenges associated with creating wound healing models
The mouse has frequently been utilized as a wound healing model, but the skin repair processes in mice are significantly different from human wound healing mechanisms.
Results of mice studies often do not provide practical insight into humans in clinical research.10 There is a demand for in vitro models that offer a more accurate representation of natural human wound healing.
In animal model systems, wound conditions are often contrived; the use of primary cells isolated from the human body could provide a more appropriate model for the study of human diseases.
Fibroblasts have been separated from chronic wounds and employed to investigate the cellular processes at work in non-healing wounds.11 These cell models offer a very appropriate model for the evaluation of chronic wounds, but they cannot provide the full picture.
Primary cell cultures are usually analyzed in isolation and so cannot demonstrate how the mechanisms in the cell type studied influence other types of cells in the nearby area.
In the complicated cascade of events that arise during regular wound healing, it is probable that fibroblasts have an inhibitory or stimulatory influence on the function of different cell types, such as keratinocytes and stromal cells.
Despite the restricted amount of data acquired from two-dimensional (2D) wound healing models, most studies still employ them because of the difficulties of effectively co-culturing a combination of unique cell types.
Normally, cell cultures are grown in monolayers and subjected to biological processes understood to be healing influences, or the cells are ‘injured’ through scraping to create a ‘wound’ that is then filled with migrating or new cells.
These cell models significantly vary from the complicated three-dimensional (3D) structure found in the physiological cellular environment.12
While some 3D models have been created, for example, electrospun fiber membranes were utilized to create a 3D scaffold for cell cultures, these require technical experience and often involve a lot of labor.13
Current wound healing models also do not account for the various stages of wound healing, and treatments could have varying outcomes according to the stage at which they are deployed.14

The presence of keratinocytes, fibroblasts, glycosaminoglycans (GAG) and collagen in all phases of the native wound healing process. Image credit: Promocell GmbH
There was, therefore, a need for a simple-to-use 3D wound healing model that facilitated the investigation of the various stages and types of cells incorporated in the biological wound healing process.
High-throughput 3D in vitro wound healing model
Magnetic 3D bioprinting has recently been used to create 3D wound healing models that can reproduce the different stages of the natural wound healing process.15 Keratinocytes and fibroblasts are magnetized utilizing biocompatible NanoShuttle™-PL14.16,17
One spheroid per well can then be produced utilizing mild magnetic forces that trigger the aggregation of cells.
Using this technique, biologically and structurally accurate 3D cellular constructs can be produced in vitro with no need for a scaffold.
The distinct stages of wound healing can be investigated in a single wound healing model by utilizing various ratios of keratinocytes and fibroblasts in different wells.
High throughput screening of the different stages of healing is also achieved utilizing a 384-magnetic ring drive.
The wound healing mechanism can constantly be observed through the use of live-cell imaging in this 3D wound healing model. CytoSMART® Omni automatically analyzes and images wound closure every hour in each well of the 384-well plate.18
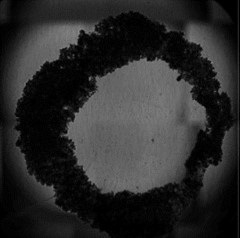
Fig. 1: (Left) A 96-well plate placed on the CytoSMART® Omni inside a humified incubator. (Right) 3D ring construct representing a dermal wound.
Along with enabling research into the development of new treatment measures to enhance wound healing, the magnetic 3D cell culture technique will assist other experimental disciplines in the field of regenerative medicine and will speed up developments in personalized cancer treatments.19,20
Summary
A high-throughput, readily-reproducible 3D in vitro wound healing model has been produced that correctly reproduces physiologic healing and is simple to use. The CytoSMART live imaging technology and Greiner’s magnetic cell culture technique are used in this model.
The creation of distinct spheroid cell constructs in different wells of a 384-well plate utilizing varying compositions of fibroblasts and keratinocytes enables the various stages to be screened with high throughput.
References
- Grice EA, Segre JA. Nat Rev Microbiol. 2011;9(4):244-253.
- Richmond JM, Harris JE. Cold Spring Harb Perspect Med. 2014;4(12):a015339.
- Slominski AT, et al. Adv Anat Embryol Cell Biol. 2012;212:v-115.
- Romanovsky AA. Acta Physiol (Oxf). 2014;210(3):498-507.
- Takeo M, et al. Cold Spring Harb Perspect Med. 2015 Jan 5;5(1):a023267.
- Huang C, Tet al. Current Problems in Surgery 2014;51(7):301-331.
- Mohd Zubir MZ, et al. Int J Environ Res Public Health. 2020 Aug 21;17(17):6103.
- Nour S, et al. J Biomed Mater Res A. 2021 Apr;109(4):453-478.
- Olsson M, et al. Wound Repair Regen. 2019;27(1):114-125.
- Zomer HD, TRentin AG. Journal of Dermatological Science 2018;90(1):3-12.
- Caley M, et al. Int J Mol Sci. 2018;19(4):1001.
- Grazul-Bilska AT, et al. Drugs Today (Barc) 2003;39(10):787–800.
- Kapałczyńska M, et al. Arch Med Sci. 2018 Jun;14(4):910-919.
- Iyer K, et al. Tissue engineering and regenerative medicine 2018;15(6):721–733.
- PromoCell application note. https://promocell.com/
- Greiner. https://3dcellculture.gbo.com/
- Tseng H, et al.. Acta Biomater. 2014 Jan;10(1):173-82.
- CytoSMART® Omni. https://cytosmart.com/products/omni
- Hou S, et al. SLAS Discov. 2018;23(6):574-584.
- Eckhardt BL, et al. PLoS One. 2018;13(5):e0195932.
About PromoCell
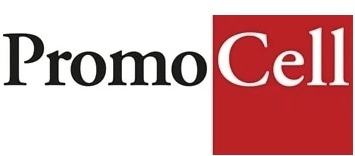
At PromoCell, we help scientists do better research with a world-class portfolio of human primary, stem and blood cells as well as optimized cell culture media. With over 30 years of expertise, we are recognized globally for supplying scientists with the tools and support they need to do groundbreaking research.
All our products comply with European biomedical conventions, ensuring human rights and donor privacy are always protected. Our ISO certifications demonstrate our absolute commitment to quality and our EXCiPACT™ GMP certification enables us to produce our cell culture media and reagents according to GMP standards as a manufacturer of pharmaceutical excipients.
Each year 600 peer-reviewed publications feature PromoCell products. We operate in over 40 countries around the world, helping scientists with all of their research needs.
Learn more about PromoCell on our website, or connect with us on Facebook, YouTube, Twitter or LinkedIn.
Sponsored Content Policy: News-Medical.net publishes articles and related content that may be derived from sources where we have existing commercial relationships, provided such content adds value to the core editorial ethos of News-Medical.Net which is to educate and inform site visitors interested in medical research, science, medical devices and treatments.