An overview of soil
Soil consists of three basic parts including plant debris, microorganisms within the soil that decompose organic matter, as well as stable organic matter that is also referred to as humus. The composition of soil ultimately determines its physical, chemical, and biological properties, which include soil aggregation, ion exchange, and nutrient circulation.
The organic carbon present within soil plays a significant role in the global carbon cycle. In fact, recent estimates suggest that soil organic carbon stocks are about twice as high in the atmosphere and three times as high in the vegetation. Depending on the climate, vegetation, and management, soil can function as either a reservoir or source for carbon.
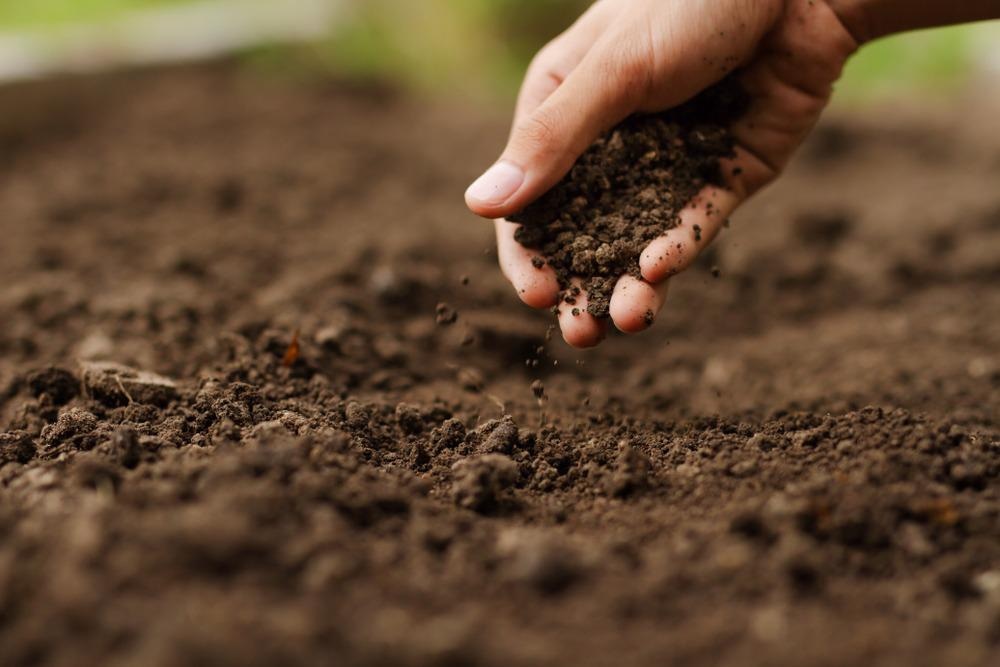
Image Credit: Piyaset/Shutterstock.com
Monitoring microbial properties of soil
Typically, fertile soil will contain a concentration of 106 to 109 bacteria per gram of soil. Aside from bacteria, microorganisms within the soil can also include protozoa, algae, fungi, and actinomycetes. These microorganisms play a crucial role in determining the texture of soil through their organic secretions, as well as maintaining the decomposition of soil organic matter, nutrient transformations, and small clay aggregation.
Due to the wide range of responsibilities that microorganisms have within the soil, many researchers are often interested in biomonitoring these microbial properties at the “biomass level.” To assess the presence of the microbial community that is present within a given soil sample, a value that is otherwise expressed as the overall biomass, researchers will often measure the amount of carbon immobilized in microbial cells (Cbio). Through this cost-effective, simple, and rapid approach, researchers are able to gain a holistic understanding of the soil’s ecosystem as well as assess the status of the microorganisms present within the soil sample.
One of the most common ways in which soil microbial biomass is calculated is through the fumigation-extraction (FE) method. This method first requires the soil microbial cells to be killed and lysed through chloroform fumigation. The two extracts that are produced through this method include fumigated soil, which contains both the intracellular and extracellular organic matter, as well as the non-fumigated portion of the soil that only contains extracellular organic matter. Typically, Cbio can be calculated as the amount of extractable carbon present within the non-fumigated sample.
Useful biochemical information can also be obtained from soil organic matter that is extracted with 0.5 molar (M) potassium sulfate (K2SO4) from non-fumigated soil samples. The product obtained through this approach represents the light fraction that can be easily degraded by microorganisms, thus representing the microbial activities and growth characteristics that are present within the soil sample.
However, various researchers often indicate that this approach is not sufficient to determine a complete ecological risk assessment or to fully evaluate the quality of the soil. To this end, these critics claim that the overall biomass value is ambiguous and does not provide a full picture of the microbial community structure or status of ongoing processes.
Pollutant detection
In addition to utilizing biochemical analysis for monitoring the health of normal soil samples, this approach can also be used to assess the effects of anthropogenic activities, particularly those that result in environmental pollution, on soil samples. Unfortunately, due to the activities of the modern industrial, urban, and agricultural sectors, toxic chemicals and metals are often released into the environment. In particular, the deposition of excessive amounts of heavy metals into agricultural soil can lead to contamination and increase uptake of heavy metals in crop plants, thus compromising the integrity and safety of any food products.
Chromium (Cr), for example, is often released into the environment through numerous industrial activities including tannery, electroplating, paints, cooling towers, and timber treatment. Although there is limited information on how Cr deposition can alter plant growth and metabolism, researchers have observed that Cr toxicity can alter the morphological, physiological, photosynthetic, and defense mechanisms of various plant species. Furthermore, Cr appears to produce reactive oxygen species (ROS), alter mineral uptake, and disrupt the balance of water within plant cells.
To mitigate the effects of environmental pollution on affected soils, phytoremediation has emerged as a highly effective tool that is inexpensive, environmentally friendly, and non-destructive to the soil. In short, phytoremediation includes the extraction, translocation, and accumulation of soil contaminants.
In addition to phytoremediation, bioaugmentation of microorganisms has also emerged as another useful biochemical strategy for detoxifying polluted soils. Through this approach, microorganisms enhance the efficacy of the phytoremediation process through a mechanism known as bio-assisted phytoremediation. Previous studies have found that this combined approach can alleviate heavy metal stress on polluted samples by increasing the production of antioxidant enzymes that can directly eliminate ROS, increase nutrient update, and much more. Recently, a group of researchers found that Bacillus xiamenensis PM14 effectively enhanced the antioxidant activities of the Sesbania sesban plant following its exposure to different levels of Cr toxicity.
Conclusion
The biochemical analysis and monitoring of soils is essential in ecological risk assessment and food safety protocols. These assays can be used to both monitor the normal physiological processes that take place in soil samples, as well as distinguish contaminated soils from those that are safe for human and animal consumption.
References:
- Wojciech, P., Ewa, B., & Jaroslaw, L. (2019). Soil biochemical properties and stabilization of soil organic matter in relation to deadwood of different species. FEMS Microbiology Ecology 95(3). doi:10.1093/femsec/fiz011.
- Apostel, C., Dippold, M., Glaser, B., et al. (2013). Biochemical pathways of amino acids in soil: Assessment by position-specific labeling and 13C-PLFA analysis. Soil Biology and Biochemistry 67; 31-40. doi:10.1016/j.soilbio.2013.08.005.
- Raju, M. N., Golla, N., & Vengtamapalli, R. (2016). Soil Microbiological Properties. Soil Enzymes; 11-15. doi:10.1007/978-3-319-42655-6_3.
- Hofman, J., & Dusek, L. (2003). Biochemical analysis of soil organic matter and microbial biomass composition – a pilot study. European Journal of Soil Biology 39(4); 217-224. doi:10.1016/j.ejsobi.2003.08.002.
- Din, B. U., Amna, Rafique, M., et al. (2020). Assisted phytoremediation of chromium spiked soils by Sesbania Sesban in associated with Bacillus xiamenensis PM14: A biochemical analysis. Plant Physiology and Biochemistry 146; 249-258. doi:10.1016/j.plaphy.2019.11.010.
Further Reading