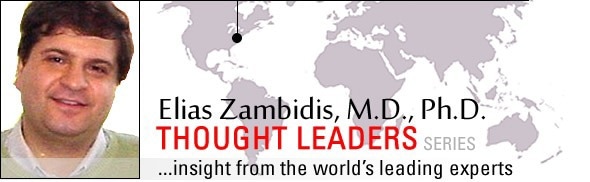
It was recently announced that you have developed a reliable method to convert blood cells to a primitive stem cell state. Please could you tell us more about this primitive state?
The primitive stem cell state we created is called an “induced pluripotent stem cell” (iPSC). iPSC are a new, unique, and artificially-created type of stem cell that were first described in 2006 by a Japanese scientist named Shinya Yamanaka. He and his colleagues first created iPSC using genetically modified mouse fibroblast cells with a method called “transcription factor-based reprogramming”.
iPSC are essentially immortal cell lines that have characteristics of embryonic stem cells that are normally derived from human embryos that are several days old (following conception).
Both embryonic stem cells and iPSC can potentially make any cell type or tissue/organ in the body. If you inject and mix a mouse iPSC line with a healthy mouse embryo, a completely normal mouse will form that is a “chimeric” mixture of both the iPSC line and the normal mouse embryo. That’s how potent these stem cells are.
In 2007, several groups around the world (including Yamanaka’s group) were successful in further using transcription factor reprogramming to generate human iPSC from human fibroblasts using the same four genetic (Yamanaka) factors his group first described (SOX2, KLF4, OCT4, and MYC). Other additional factors were also later used by others to create human iPSC.
Today, there are scores of labs all around the world, including my own at The Johns Hopkins University School of Medicine, that can routinely generate human iPSC using Yamanaka’s general genetic engineering approach. The method is extremely tedious and time-consuming, but highly reproducible with the proper scientific training and experience.
Please could you give a brief introduction to blood cells and what type your research was on?
Blood is composed of red cells (erythrocytes), white cells (myeloid cells, lymphocytes, and rare blood stem cells), and platelets (which mediate clot formation during bleeding). Red cells and platelets do not have nuclei and DNA, and therefore cannot be genetically engineered.
In this study we specifically focused on the ability of the white myeloid cell population to be efficiently converted into iPSC without the use of viruses. The use of viruses as tools to express the Yamanaka factors and to generate iPSC is currently a routine practice in the field due to its ease of use. Yet, this approach has the potential to create cancerous cells that may make the iPSC not clinically useful later on.
The type of white cell we used can be easily obtained either from a blood draw, or with a needle under local anesthetic from the hip’s bone marrow. Additionally, they can be obtained from umbilical cord blood samples harvested at a baby’s delivery.
Blood stem cells are extremely rare in the bone marrow, and even rarer in peripheral blood. However, our study used the more abundant white cells that are young, but are not quite the rare stem cells.
In our studies, these abundant young white cells (progenitors) were obtained from various normal human sources (e.g., cord blood, adult peripheral blood, and bone marrow blood).
Most of our human iPSC experiments focused on the cord blood myeloid progenitors to demonstrate proof of principle. We used a blood progenitor marker called CD34 to isolate these white cells from the various sources, and then we let these cells expand and mature in a culture dish with bone marrow “helper” (stromal) cells before we genetically converted them into human iPSC.
Although we did this in a laboratory dish in defined artificial conditions using the “helper” stromal cells, these white cell progenitors normally expand and mature naturally with these stromal cells in your bone marrow to make more specialized white cells called neutrophils, monocytes, and macrophages, which fight off bacterial infections that may enter your blood from a cut or scrape.
Importantly, your body contains billions of such white cells, and thus represent a highly accessible source of adult cells that could be used to generate human iPSC using our method. The more primitive, but rarer blood stem cells have further abilities to make red blood cells, lymphocytes (immune cells), and platelets.
Our highly efficient method does not require the use of purified rare blood stem cells, and this is a big distinction in our approach, since others had previously suggested you needed to use the rarer stem cells to efficiently generate blood-derived human iPSC. We showed that this is not so.
It should also be noted that the cord blood cells we used are normally discarded at delivery, and yet represent a valuable resource for research and future clinical therapies.
Cord blood cells also have many other unique advantages over adult blood cells. For example, because they are younger, they contain less acquired genetic problems with them. Moreover, there are organized blood banks for cord blood cells all around the world that could potentially be used to create a bank of hiPSC using our efficient method. Unpurified cord blood cells or adult bone marrow can also easily produce the “helper” (mesenchymal stromal) cells we used to boost our stem cell conversion efficiency.
Thus, all the ingredients you need to efficiently create a patient-specific stem cell without a virus can easily be obtained from the same patient’s blood draw with our approach. These patient-specific human iPSC lines can then be used to generate genetically-matched tailored heart cells, brain cells, pancreatic cells, or vascular cells to treat various diseases ranging from heart attacks to diabetes.
What is the advantage of developing a method to efficiently convert blood cells to a primitive stem cell state?
As described above, human iPSC are created by introducing defined genetic factors into fully differentiated cells obtained from an adult person’s body (e.g., skin cells or blood cells). This is usually done by using a genetically engineered virus to express the defined factors. It is a tedious and extremely inefficient process. Only rare (<1%) fractions of the genetically engineered cells actually convert into a successful iPSC line. Moreover, of the rare iPSC lines that do form, the majority are of a terrible quality, and often cannot efficiently make the cell types one wants.
In general, many groups have shown that many of the human iPSC lines that result from this process are not as good as human embryonic stem cells in doing the job of generating all the cell types of the body. Many iPSC lines seem to retain a memory of their original cell type, and some investigators believe that this may handicap their ability to make all the cell types well. This is called “epigenetic memory”. Thus, there is a need in making iPSC with greater ease, without viruses, and with higher quality and little epigenetic memory.
Moreover, most iPSC made with viral methods have been shown to have the potential to convert into cancerous cells at a later time. Viral expression of the genetic factors that convert an adult cell into a pluripotent stem cell causes a permanent integration of them into normal chromosomes, and this can cause malignancy later. This is because the Yamanaka factors which help convert an adult cell into a pluripotent state, can also behave as oncogenes when expressed abnormally.
Thus, there is a large effort in many labs worldwide to figure out how to make iPSC without the use of viruses, or without permanent integration of the Yamanaka factors. However, this has turned out to not be an easy task. Several groups have reported some success, but it appears to be more inefficient to turn adult cells into iPSC without the use of these viral methods.
Our lab, as well as several others around the world recently appreciated that blood cells possess distinct advantages in their potential for being converted into iPSC. Last year, my group and two other groups simultaneously and independently reported that early blood cells found in cord blood or bone marrow are fantastic targets for making human iPSC without viruses. To do it, we all used nonviral DNA “episomal” constructs to temporarily express Yamanaka’s factors into these early blood cells. The efficiency was pretty good for all of us compared to using skin cells, but it was still quite low (0.1%-1%).
These episomal DNA constructs can be forced into the nucleus of blood cells with an electrical pulse. It is tricky getting them in there though because the DNA constructs are very large, and they barely fit to pass through the holes we punch into the cells with our electrical pulse. Once in the cells, our DNA constructs express the Yamanaka factors for short periods of time (several weeks), and then they degrade naturally without leaving a genetic footprint that they were there.
Although many others had suspected a “specialness” of blood cells for converting into human iPSC, our study just published was the first to optimize and exploit the special potential of myeloid cells so that we were able to convert the majority of the blood cells that got our DNA construct into a pluripotent state.
What had previously prevented scientists from converting blood cells back to a primitive embryonic stem cell-like state?
In recent years, there has been an explosion in the study of iPSC biology. Scientists from some of the best and brightest labs around the world are working with them. Many outstanding groups have now invested much time and resource in solving the frustrating technical caveats of Yamanaka’s imperfect yet amazing method.
These efforts have included:
- developing method to generate human iPSC more efficiently without viruses
- developing methods to make human iPSC with a higher quality that is equivalent to bona fide human embryonic stem cells
- further developing methods to coax human iPSC into efficiently generate clinically desired replacement cells (e.g. brain cells, heart cells, blood cells, etc)
Despite the great potential of human iPSC, there are currently important technical problems that have prevented scientists from achieving these three goals. For example, there is an ongoing debate on what is the most optimal and most reliably accessible cell type that can be easily obtained from a patient for genetic conversion into a high quality iPSC line without viruses.
Skin cells, hair cells, fat cells, and blood cells have all been proposed as ideal “donor” cell sources because of their abundance. Blood cells and skin cells are especially attractive since you can easily get a skin biopsy or a blood draw in a doctor’s office to convert into your own tailored human iPSC line. Our study shows that nonviral conversion of skin cells into human iPSC is nowhere near as efficient as the myeloid cells.
How did you manage to develop a way for converting blood cells into a primitive stem cell state without using viruses?
In our manuscript, we demonstrated for the first time that contrary to previous suggestion, it is indeed possible to generate human iPSC without viruses with an extremely high efficiency in a very large fraction of factor-expressing human myeloid cells. The efficiency of converting myeloid cells into human iPSC with our approach was >10,000 times more efficient than with skin cells.
We used an optimization that employs the natural stromal micro-environment of these myeloid cells. Extrinsic niche signals that were provided by the mesenchymal bone marrow stromal cells (BMSC), as well as synergistic stimulation from hematopoietic growth factors we put into our system was sufficient to convert a great majority of the myeloid cells to human iPSC with remarkable rapidity.
This dual stimulation significantly boosted the ability of myeloid cells to convert to iPSC, and only a single and simple DNA episome construct that expressed the four Yamanaka factors transiently was needed. The conversion was rapid and complete in only 1-2 weeks.
The DNA was subsequently degraded away, and was not permanently integrated after the conversion had occurred. Thus, these non-integrated human iPSC were immediately ready for research and preclinical studies.
In our studies, we found that our stromal priming method was so efficient in converting the myeloid cells into human iPSC that the rate-limiting step for this conversion was mostly the poor efficiency of just getting our large, bulky DNA construct into the blood cells. When we enriched for only the blood cells that had successfully received our DNA construct cargo, and then measured the efficiency of iPSC conversion in this selected group, we discovered that we were actually getting unprecedented efficiencies of at least 50% conversion into high quality human iPSC.
What are the advantages of using these converted blood cells rather than human embryonic stem cells that have similar properties?
Human iPSC have very similar characteristics and differentiation potential with the human embryonic stem cells that can be obtained from an in vitro fertilization (IVF) clinic, and that are harvested from a 5-6 day multi-cellular human embryo.
However, although human iPSC mimic human embryonic stem cells and are very, very similar in many respects, they are indeed not completely equivalent genetically. There have now been described some unique genetic and epigenetic differences between them that may make them harder to differentiate to the right cell types.
Yet, both human embryonic stem cells and iPSC have theoretically equal potential to make any type of cell in the body if we can find ways to make them equal in quality. Most importantly, because human iPSC are derived from a patient’s blood cell with our method, we completely bypass the controversy of using discarded human embryo cells for stem cell therapies.
What impact do you think your research will have?
- We believe that the inefficiency problem of generating non-viral, clinically useful human iPSC is essentially solved if one uses myeloid cells and our stromal co-culture method. Our ongoing work has also recently revealed that these rapidly-reprogrammed BMSC-primed nonviral CB-iPSC also have more rapid erasure of epigenetic memory marks, and have much more robust differentiation abilities compared to other human iPSC made with other methods.
- The very high efficiencies for converting myeloid cells into human iPSC may now open up the mysterious steps of factor-based reprogramming to more detailed biological studies. The cellular biology of this process for human cells can now be looked at more accurately, since synchronized populations of converting cells can now be collected at various time points. These results may also open new avenues of research for elucidating the micro-environmental factors that drive efficient reprogramming in other donor cell types like skin cells and hair cells.
What plans do you have for further research into this field?
One of the most important take home messages from our published paper was that efficient induced pluripotency required a coordinated activation of common stem cell gene networks that regulate self-renewal and differentiation in both hematopoietic progenitors and embryonic stem cells.
We are currently seeking to learn what activated blood cells can teach us about the pluripotent stem cell state. We do not think that it was an accident that blood cells were so easily converted to human iPSC compared to skin cells in our studies. We believe that there is a very important and interesting biology to uncover here.
Interestingly the gene networks that were expressed in the activated myeloid cells, and that facilitated conversion to iPSC are also involved in turning normal cells into a cancerous state in other contexts. Thus, we are interested in investigating what regulates the balance between turning an adult cell into a “normal” iPSC, or when this process goes awry, into a malignant cell.
We are also keen in elucidating exactly how the bone marrow stromal signals we used were able to further rev up this whole process of turning a myeloid cell into an iPSC line. Finally, we are currently studying the differentiation potential of these unique blood-derived human iPSC to make heart cells, vascular cells, retinal cells, and brain cells.
Would you like to make any further comments?
The ability to convert a differentiated blood or skin cell back into an embryonic state is nothing short of revolutionary. This phenomenon has wide impact not only for regenerative medicine, but also for the future understanding of the biology of aging and cancer.
This field is moving at a remarkably fast pace. It as an exciting time to be both a scientist and a physician, and to witness remarkable developments in this field unfold literally almost every week in the scientific journals. The quality of the science that is being conducted is very high, and impressively collaborative.
Scientists of various interests and fields are contributing. Those of us working in the field are all reading and learning from each other’s papers, and then rushing to reproduce each other’s findings and take it to the next stage. With every scientific step forward in iPSC biology, I have found that another scientist from another group will quickly pick up the baton, and then publish the next logical advance in this puzzle in only several months.
Rapid, open access publication of each other’s important discoveries has facilitated this process of advancement. In my opinion, we are all in the midst of a remarkable scientific revolution with a wide and exciting impact for medicine.
Where can readers find more information?
The published paper by Park et al (2012) can be found at this link: http://www.plosone.org/article/info%3Adoi%2F10.1371%2Fjournal.pone.0042838
The Zambidis Lab website: https://www.hopkinsmedicine.org/
For more general information about induced pluripotent stem cells: https://stemcells.nih.gov/info/basics
Funding of this research
This work was supported by grants from the National Institutes of Health, and the Maryland Stem Cell Research Fund.
About Elias Zambidis, M.D., Ph.D.
Dr. Elias Zambidis is an Assistant Professor of Oncology and Pediatrics at the Johns Hopkins School of Medicine.
Dr. Zambidis practices Pediatric Oncology in the Dept of Oncology, Kimmel Comprehensive Cancer Center at Johns Hopkins, and is the Principal Investigator of a laboratory in the Stem Cell Program at the Johns Hopkins Institute for Cell Engineering.
Dr. Zambidis earned his M.D./Ph.D. in the Medical Scientist Training Program (M.S.T.P.) at the University of Rochester, Rochester, N.Y. He did his Pediatrics Residency in the Department of Pediatrics, Washington University, St. Louis, Missouri, and his clinical/research fellowships in Pediatric Hematology/Oncology at the Johns Hopkins Hospital and the National Cancer Institute at the NIH.
His clinical expertise is in pediatric oncology specializing in hematologic malignancies, developmental hematopoiesis, blood and bone marrow transplantation (BMT), and stem cell biology and therapeutics.
Dr. Zambidis is interested in the developmental biology of normal and malignant hematopoietic stem cells. He uses genetic manipulation and differentiation of both embryonic and adult pluripotent stem cells to study the cellular and molecular mechanisms of human hematopoiesis.
Using human embryonic stem cells (hESC) as well as induced pluripotent stem cells (iPSC), he is exploring whether a human hemangioblast (bipotential progenitor of hematopoietic stem cells (HSC) and endothelium) gives rise to the entire human hematopoietic system, and whether these cells can be derived and expanded for vascular and hematopoietic disorders.
His laboratory is studying the role of a variety of proteins and signaling molecules that are critically important in orchestrating the initiation of human embryonic hematopoiesis. hESC-derived blood progenitors are important in the understanding of the developmental origins of pediatric leukemia, but also for clinical HSC transplantation.