Interview conducted with Professor Björn Wängler, Professor for Molecular Imaging and Radiochemistry from Heidelberg University.
Could you provide a brief introduction to yourself and your role?
I’m Björn Wängler, Professor for Molecular Imaging and Radiochemistry at the medical faculty Mannheim of Heidelberg University. I’m a radiopharmaceutical chemist by background and completed my PhD in 2004 at the University of Mainz.
Two years ago I started collaborating with Bruker and we installed a new Xtreme system, the Albira PET/SPECT/CT and one Tesla MRI system.
We have a lot of research collaborations, including that with Bruker. We have the “Industry-on-Campus” collaboration – and the so called functions campus M²OLIE. We work together with companies like Bruker, Siemens and some others. It's all about oligometastasized patients. We want to try to develop new techniques to treat and diagnose them and this is one of the research projects.
Another one is EU funded. It's called MITIGATE, which is a project focusing on rare diseases. We also develop new radiopharmaceuticals for the treatment and diagnosis of resistant GISTs (gastrointestinal stromal tumors).
There are essentially three aspects to our research. The first aspect is performing easier labeling, both with fluorescent dyes and with radionuclides, to make it more convenient like it is for SPECT radiotracers. So, you have some kind of a kit, a radiochemistry system. Another aspect is, of course, the development of new target-specific structures.
The third aspect is the design of a molecule for diagnosis or treatment because we are also doing endoradiotherapy with radionuclides. We have collaborations around this and one is a very important collaboration with the Central Institute of Mental Health. We are doing addiction research there. We used FDG in the cocaine addictive rat models.
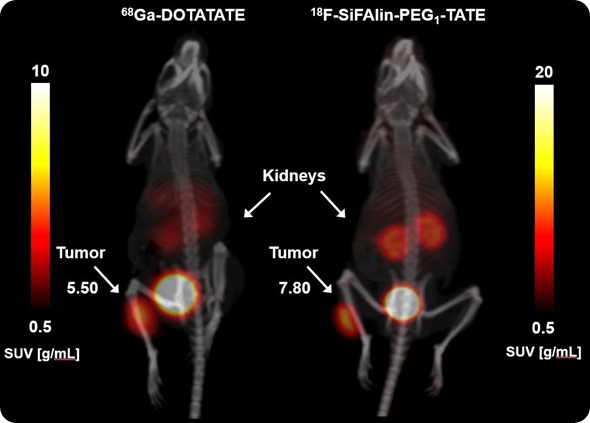
Another one is the detection of cancer in a gastric cancer model, together with the second medical department here at the University Hospital. Our own research was designing the molecules by multimerization of target structures and then the tests in a mouse tumor model.
Our research is primarily on new molecules for cancer diagnosis and treatment and the design of new target structures, especially for prostate cancer and breast cancer. These are our main focuses at the moment, but there are also some others.
What is the impact of your research on the medical field?
We have a very close collaboration with the clinical departments. I'm also involved as a radiochemist and radiopharmacist in the clinical department here and we are developing new radiotracers that can be used directly in a clinical setting.
In Germany, you only need approval from the ethics committee along with some basic toxicology work, so the process is sometimes a bit simpler than in other countries. Essentially after three to four years of research and toxicology studies, you can use the radiotracer directly in a clinical setting, at least with one or two patients and see if it works in the human setting. It's a direct chain from bench to bedside in our department because we have the chemical labs, then the in vivo labs and then the clinical department.
Also it is relatively simple for our collaborators in the medical physics department to calculate if a special new radiotracer for diagnosis or therapy can also be useful in the clinical setting. This is partly because the instruments are essentially the same, i.e. PET/CT, thus it is easier to predict if a new radiotracer be useful not only in the animal but also be beneficial for the patient.
What is the importance of instrumentation in your research?
It's mainly about having this direct effect; this direct calculation. We have a collaboration partner, Professor Glatting here in the Medical Physics Department who calculates the radiotracer pharmacokinetics and what makes the in silico prediction of the pharmacokinetics of a new radiotracer possible for humans and he absolutely needs the in vivo data for these calculations. He needs the curves and not only the time points.
Of course, if you have such instrumentation, you also do not need as many animals because if you do ex vivo studies where you have to sacrifice the animals in order to take and measure the organs, then you need more animals. It's not so precise and you cannot really predict whether that radiotracer would be useful in a clinical setting. It might be enough for a publication, just for a radiochemistry publication, but to really have the chain from bench to bedside, you need the instrumentation.
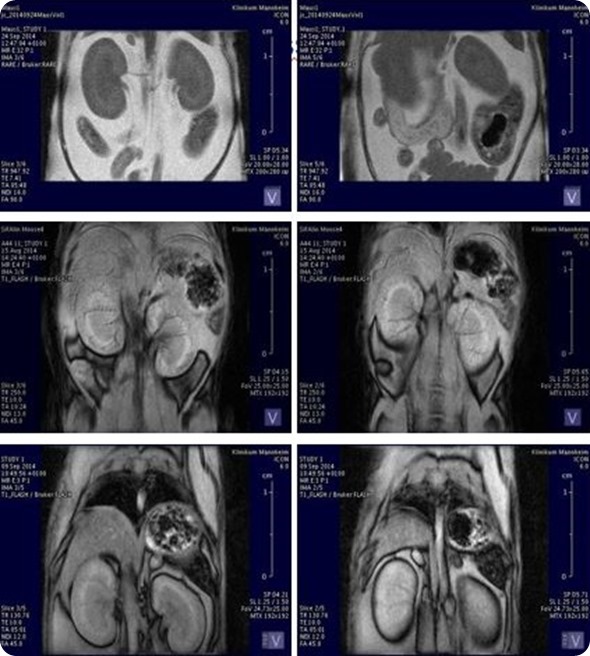
ICON: Organ volumetry and tissue characteristics
I know you have a MRI, a PET/SPECT/CT and the optical imaging. Can you just discuss very briefly what each instrument gives you and how they work together?
Our name is Molecular Imaging, but in 90% of all cases, we are working on radiochemistry research and the development of new radiotracers. Therefore, the most important instrument for us at the moment is our Albira system.
We're also doing a little bit of research with new fluorescent dyes that will be used in the in vivo Xtreme system. Our workhorse is the Albira system but as we also have a collaboration with neurology, and especially with the psychiatry department, we also need the one Tesla MRI. We need an MRI to obtain better contrast in the brain.
It would really be great to have a PET/MRI, but we are pretty happy with the systems we have at the moment. We have a multimodal bed, so after the PET scan, you can just place the animal with that full bed into the MRI scanner.
At the moment, we don't have a cyclotron on site and therefore it would be really great if we had injection systems for more than one animal at one time point. It would be good to have a perfusion pump for three or four animals because the Albira can measure three or four animals at once. If we had a perfusion pump for that, that would make things easier and more convenient for us. Apart from that, I think we are pretty happy with our instrumentation.
What are your future research plans?
We're aiming to actually have real multimodal contrast agents that can be used not just for one application, so for PET or for MRI or for fluorescent imaging, but a combination of applications.
Of course, it’s a problem to use optical imaging in the patient setting because you can only see one or two centimeters deep into the tissue. However, it could have potential in minimally invasive treatments, if you have a substance which is labeled with a fluorescent dye and could be labeled with a radionuclide, then you could use this same molecule in an in vivo non-invasive setting.
You could perform your PET scan and then afterwards, bring the patient into the operating room. Then, the surgeon can see the fluorescent dye with his camera. He would have a camera in his minimally invasive endoscope and be able to see not the radiotracer, but the fluorescent dye on the decayed radiotracer.
Another point, would be the use of gold nanoparticles. Gold can very easily be activated by neutrons and therefore you have a scaffold for derivatization on the surface of this gold nanoparticle. You could also activate a particular gold nanoparticle with neutrons and then you would have something that could be useful in endoradiotherapy. You would be treating the patient with the radionuclide directly.
Can you tell us a little bit more about how your research impacts the medical field?
We have a close collaboration with the medical department here, especially with the radiology and nuclear medicine departments. I'm also involved there as a radiopharmacist and I'm responsible for the development of new radiopharmaceuticals that are used in a clinical setting.
Therefore, we’re in a great position here to cover all the strains starting from the lab bench, developing a new molecule with organic chemistry and then test the properties of this radiotracer in vitro. Then, we can move on to the pre-clinical in vivo experiment and then go into the patient.
From what I have heard, it's a little bit simpler than in other countries to get a new compound into the patient. Essentially, you need the okay from the ethical committee and radiation authorities and then you have to do some not so complicated toxicology studies about your new radiotracers. Then, you usually get the allowance to test that in at least one or two patients and prove that it is really useful in a clinical setting. Then you can further evaluate its clinical benefit in more patients.
We have a close collaboration and it really impacts the research. It's not only research for scientific journals. That was my problem when I started my career. At some point I thought, “okay, I'm a chemist and I'm producing results, but nobody really cares.” Now, my work may be published in a scientific journal or introduced into a book chapter, for example, but does someone really care about that? No, not really.” Then I switched to radiopharmaceutical chemistry and that was really my field because you have real impact.
Can you share an example of research you've done in the past that you've been able to implement?
There's one molecule we have tested within the last five years in the pre-clinical setting and we did a lot of development that's essentially about easier radiolabeling. I talked about this kit system that could be used to produce radiopharmaceuticals.
You just take your radionuclide, inject it into a glass vial with your precursor and wait for five to ten minutes. Then you have your radiotracer. This is something we developed for peptides and it will be used in the first one or two patients within the coming weeks.
How many researchers do you have working here with you?
We have ten at the moment. Two to three are working on in vivo imaging and pre-clinical in vivo imaging and the others are pharmacists and chemists. There are some collaborations with some physicists who work here and they are doing very well.
Can you tell us a little bit about your collaboration with Bruker?
We established the Bruker Imaging Reference Center here together with colleagues in Ettlingen which is 75 kilometers away. We have a very close collaboration that is not only about being a reference center, but also about research.
Bruker are involved in the research campus initiative M²OLIE and they are working together with us to make the instrumentation better and to help us if we have some minor problems with a machine. So, it's a really good and close collaboration that’s fruitful, I think, for both sides.
We can test the instruments and methods under real lab conditions so Bruker can learn how the instruments will perform in the real world, not just in a R&D setting. As part of the collaboration we also test new software and get to work with the applications team.
Can you tell us a little bit about the types of instrumentation that you're using and what kind of information you're getting from each?
In the in vivo Xtreme and the Bruker Albira PET/SPECT/CT, it's the pharmacokinetics of our new radiotracers or for our new fluorescent dyes coupled to target structures.
In the case of the MRI, it's mostly a combination with the PET system, which allows a better contrast in the brain or body and enables us to see the soft tissues better or to differentiate better between them in the body.
If there was a dream instrument that you could have, what would it be?
At the moment, a PET/MRI, with a three-Tesla MRI and PET system, where we only have to inject some megabecquerels of the compound and that has a resolution of 0.5 millimeters. This would give us higher resolution images.
About Bruker
Bruker is market leader in analytical magnetic resonance instruments including NMR, EPR and preclinical magnetic resonance imaging (MRI). Bruker's product portfolio in the field of magnetic resonance includes NMR, preclinical MRI ,EPR and Time-Domain (TD) NMR. In addition.
Bruker delivers the world's most comprehensive range of research tools enabling life science, materials science, analytical chemistry, process control and clinical research. Bruker is also the leading superconductor magnet and ultra high field magnet manufacturer for NMR and MRI solutions.