Electronic wearable patches have been devised to monitor various health conditions by noninvasively detecting biomolecules on the skin surface.
A new Nature Communications study discusses the development of novel skin patches capable of deep detection of biomolecules, which correlate better and more rapidly with physiological states. For example, the photoacoustic patch described by the researchers, who are engineers at the University of California San Diego, can produce a three-dimensional (3D) map of deep tissue hemoglobin.
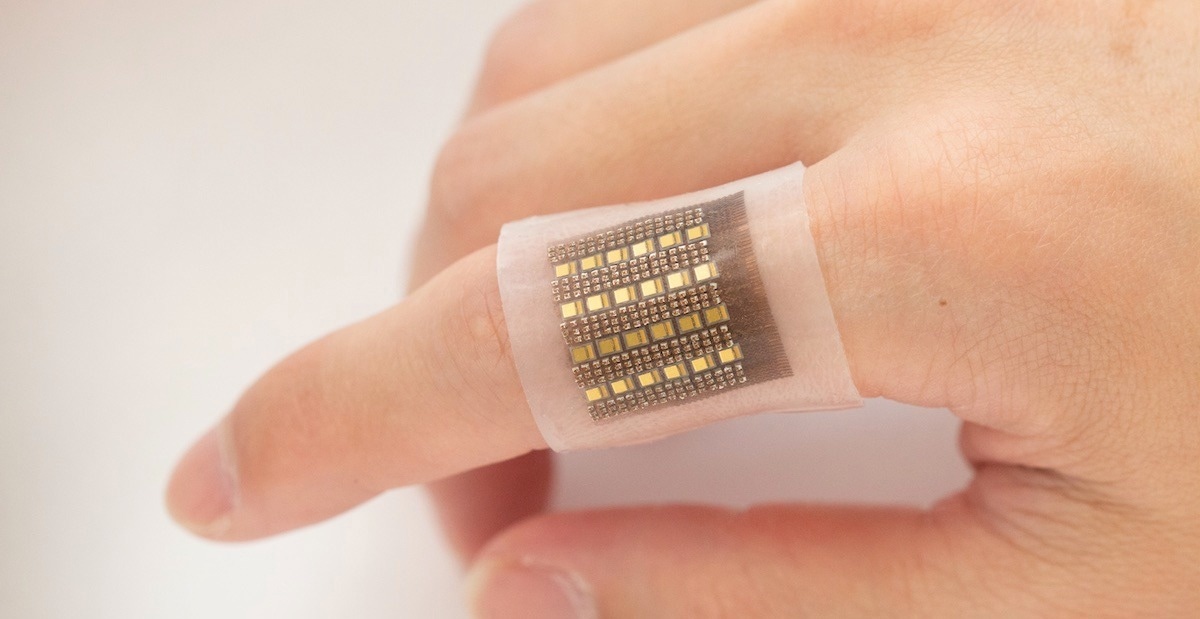
Image - A photoacoustic sensor could help clinicians diagnose tumors, organ malfunctions, and more. Photo by Xiaoxiang Gao for the Jacobs School of Engineering at UC San Diego.Study: A photoacoustic patch for three-dimensional imaging of hemoglobin and core temperature. Image Credit: MDGRPHCS / Shutterstock.com
With access to biomolecules in deep tissues, this technology adds unprecedented capabilities to wearable electronics and thus holds significant implications for various applications in both basic research and clinical practice.”
Introduction
Biomolecules in the body can be used to monitor health and disease states and assess the outcomes of certain treatments. The amount and location of hemoglobin in the body, for example, is key to determining how well various tissues and organs are perfused and the accumulation of blood in that area.
Hypoperfusion is a potential cause of severe organ dysfunction and often occurs during myocardial infarction, vascular diseases of the limbs, or post-transplant. Conversely, congestion with blood is observable in some cancers, traumas, or inflammation.
Current methods of monitoring these states are costly, relatively inaccessible, or require sophisticated equipment or radioactive tracers like magnetic resonance imaging (MRI) or positron emission tomography (PET). Furthermore, these tools do not allow for long-term monitoring of biomolecule levels.
The current study utilizes previous advances in wearable skin patches that allow for the sensing of biomolecules through electrochemistry for deep tissue applications.
What does the study show?
The photoacoustic patch comprises several different ultrasonic transducers that detect acoustic waves and vertical-cavity surface-emitting laser (VCSEL) diodes. Both types are integrated on a common substrate composed of an elastomeric matrix and are linked to each other by coiled metal electrodes.
Low blood perfusion inside the body may cause severe organ dysfunctions and is associated with various ailments, including heart attacks and vascular diseases of the extremities. At the same time, abnormal blood accumulation in areas such as the brain, abdomen, or cysts can indicate cerebral or visceral hemorrhage
The photoacoustic patch used here has high mechanical and electrical strength and does not need to be pressed onto the skin for its attachment. Furthermore, this patch is soft but rigid at each transducer element and VCSEL diode.
The high-power VCSEL diodes generate pulses of laser beams that penetrate up to two centimeters (cm) into living tissues. The high-power VCSELs also have a high signal-to-noise ratio (SNR). Their preferred wavelength is 850 nm due to excellent deep penetration that allows deep human tissues to be probed.
This wavelength is consistent with the dominant optical absorption coefficient of hemoglobin. VCSEL diodes at this wavelength are commonly available due to low attenuation in fibers, with silicon diodes at 850 nm often inexpensive and broadly employed.
With its low-power laser pulses, it is also much safer than X-ray techniques that have ionizing radiation.”
The laser pulses excite hemoglobin molecules to produce acoustic waves detected by the transducers and then processed. When hemoglobin converts the pulsed optical energy into mechanical energy in the form of photoacoustic waves, photoacoustic signals are generated.
The transducers comprise a piezoelectric layer backed with cured silver epoxy. This material has a high energy conversion efficiency and excellent electrical conductivity that also dampens excessive vibrations to provide improved axial resolution and signal bandwidth.
The loss of detection sensitivity of the transducers is less than -10 dB, thus indicating that the patch has high imaging depth and uniform sensitivity. This allows for a detailed, high-resolution, and 3D map of hemoglobin to be produced.
Though VCSELs produce significant heat, the patch used here did not become uncomfortably hot. Instead, it became slightly hotter than the skin.
The heat generated by the patch does not exceed that of ultrasound-phased arrays and increases in incident intensity to only 4% after one hour of continuous operation. This emphasizes the stability of the VCSELs and compliance with safety standards.
Despite the significantly lower optical intensity using VCSELs compared to a conventional laser, the averages of photoacoustic signals are used for a higher SNR, leading to a lower frame rate. This is balanced by using appropriate averaging times.
[This photoacoustic imaging] takes advantages of the unique absorption characteristics of biomolecules and highly penetrating acoustic waves to achieve high spatial resolution mapping of biomolecules in deep tissues.”
Unlike ultrasound imaging, which uses acoustic impedance differences to differentiate tissues that can often lead to unclear borders, photoacoustic images are based on optical absorption contrast, which allows blood to be distinguished from other biofluids.
Simultaneously, this approach differs from currently available photoacoustic imaging systems due to the absence of complex structures and its ability to operate at ordinary temperatures. This could potentially allow imaging of people in motion.
Core temperature should be kept at around 37 °C, with a variation of 1 °C over the day. This is achieved by thermoregulation; however, a failure of this process could lead to life-threatening consequences. Earlier attempts to monitor core temperature noninvasively use longer processes limited to surface sensing.
The linear correlation between the photoacoustic signal amplitude and core temperature in the patch described here allows the temperature to be accurately and rapidly mapped during various clinical and research situations. However, since this was performed in ex vivo experiments, future studies that perform interventional calibration are needed to validate the ability of the patch to monitor the core temperature in the human body.
In vivo monitoring attempts were successful, with highly sensitive detection of blood vessels and good contrast; however, motion artifacts are possible.
What are the implications?
The electronic patch described here is unique in its design, fabrication, and working principle compared to any other wearable electronic patch. Furthermore, this novel patch was tested ex vivo and in vivo with good results that will likely allow researchers in the future to monitor the flow of blood and proliferation of blood vessels in a range of conditions, including blood vessel diameter, when vascular diseases are suspected or known to be present.
Many other biomolecules can be detected using this technology by adjusting the laser wavelength according to the biomolecule of interest. Diodes of multiple wavelengths could be incorporated to allow a more extensive range of biomolecules to be detected with increased accuracy by their absorption properties at different wavelengths.
Further work is needed for greater detection depth, which is particularly important for cardiac imaging. For these applications, VCSELs of higher power are needed for these applications to increase the SNR.
Other challenges must also be met, including calibration and smaller control electronics, rather than the bulky backend system used to acquire and process signals. These changes could allow a wearable system to be developed for measurements of hemoglobin and core temperature in deep tissues in a fast noninvasive manner.
Journal reference:
- Gao, X., Chen, X., Hu, H., et al. (2022). A photoacoustic patch for three-dimensional imaging of hemoglobin and core temperature. Nature Communications. doi:10.1038/s41467-022-35455-3.