Selected ion flow tube mass spectrometry (SIFT-MS) greatly simplifies quantitative analysis of volatile nitrosamine impurities in drug products. It provides a three-fold throughput advantage and sample prep benefits over chromatographic methods.
The known or suspected mutagenicity of various N-nitrosamines, especially N-nitrosodimethylamine (NDMA) and N-nitrosodiethylamine (NDEA), means their presence in any product involving human exposure is a concern.
Even though these compounds are commonly found in beverages, water, and foods, their discovery in sartan medicines in 2018 surprised the pharmaceutical industry.1
Elastomeric sources of N-nitrosamines, commonly employed in various packaging components, are strictly controlled since recent improvements to the manufacture of elastomers in response to historic industrial problems.2
Investigations have demonstrated that most issues with N-nitrosamine result from nitrosating agents employed during synthesis, particularly in the presence of secondary amines.3
After significant industry investigation and consultation, the United States Food and Drug Administration (FDA) and the European Medicines Agency published suitable intakes.4 Table 1 lists the limits for the most volatile N-nitrosamines.
Table 1. Volatile nitrosamines of concern to the United States FDA and EMA, with acceptable intakes for each regulatory agency. Source: Syft Technologies

As the suitable intakes are in the low nanogram per day levels, highly selective and sensitive analytical methods are required. These are commonly based on gas or liquid chromatography, using long analysis times and very complex sample preparation requiring dissolution and centrifugation.
Off-line methods are also available. They take a comparatively long time to produce results for the first sample, partly due to calibration and a comparatively low total sample throughput.
SIFT-MS greatly streamlines the sample preparation process. The drug product is weighed into the vial. The process eliminates the need for a slow chromatography step by directly applying soft chemical ionization to gas-phase and headspace samples.
The approach accelerates analysis times and considerably reduces other sample preparation, as derivatization of the highly polar nitrosamines is unnecessary.5
Due to its highly selective and sensitive nature, the headspace SIFT-MS is suited to quantitative screening analysis of volatile nitrosamines in drug products. The technique also provides several significant advantages compared with common chromatographic analysis, thanks to the fast capture of results and higher sample throughputs (see Figure 1).

Figure 1. Headspace SIFT-MS enables high-throughput screening of volatile nitrosamines, providing enhanced quality control and quality assurance. Image Credit: Syft Technologies
Method
The SIFT-MS technique
A Syft Technologies Voice200ultra SIFT-MS instrument, operating on helium carrier gas, was employed for the study discussed in this article. SIFT-MS, shown in Figure 2, used soft chemical ionization to produce mass-selected reagent ions that rapidly react with and quantify volatile organic compounds (VOCs) down to part-per-trillion concentrations (by volume, pptV).6

Figure 2. Schematic diagram of SIFT-MS – a direct, chemical-ionization analytical technique. Image Credit: Syft Technologies
Commercial SIFT-MS instruments incorporate up to eight reagent ions (H3O+, NO+, O2+, O-, OH-, O2-, NO2- and NO3- ), acquired from a microwave discharge in air.7
While these reagent ions do not react with the main components of air (N2, O2, and Ar), they effectively respond to VOCs and other trace analytes in well-controlled ion-molecule reactions. This reaction enables direct, real-time analysis of air samples at trace and ultra-trace levels without requiring pre-concentration.
High-speed switching between reagent ions provides excellent selectivity as the multiple reaction mechanisms enable independent measurements relative to each analyte. The multiple reagent ions often remove uncertainty from isobaric overlaps in mixtures that contain several analytes.
Automated multiple headspace extraction (MHE) analysis was performed on a SIFT-MS instrument alongside a multipurpose autosampler (MPS Robotic Pro, GERSTEL; Mülheim, Germany). GERSTEL’s Maestro software was utilized to control the autosampler. Samples were incubated in a GERSTEL agitator for 30 minutes at 60 °C.
Headspace was sampled using a 2.5 mL headspace syringe (heated to 150 °C). It was then injected at a flow rate of 100 μL s-1 into the SIFT-MS instrument’s autosampler inlet (heated to 150 °C) through a self-sealing GERSTEL septumless sampling head.
The nominal sample flow into the SIFT-MS instrument is 370 µL s-1. A make-up gas flow (ultra-high purity nitrogen) was also passed through the sampling head. This dilution was accounted for in the calibration curve and produced under the same conditions as those used to measure the samples.
Each sample was analyzed for 100 seconds (see Figure 3), and the concentrations reported were the average values acquired during injection (between 40 and 60 seconds). It should be noted that no internal standard was applied.8

Figure 3. Example headspace injection with synchronous SIFT-MS analysis of NDMA at 2.6-ng spike level (10-mL sample vial). Image Credit: Syft Technologies
SIFT-MS analysis of NDMA and matrix effects
The information detailing the SIFT-MS analysis of various volatile nitrosamines has been published.9 Table 2 outlines the reaction chemistry of the target analyte in this study, NDMA.
The predominant measure of SIFT-MS sensitivity is the reaction rate coefficient (k), which is greater for nitrosamines than most VOCs due to their high polarity.
Table 2. SIFT-MS reaction chemistry (rate coefficient (k), product ion formulae, branching ratio (BR as %), and mass-to-charge ratio (m/z)) for NDMA and N,N-dimethylformamide (DMF), a potential interferent. Ions in gray are not ordinarily used for quantitation or identification due to their low BR (reduced sensitivity). Source: Syft Technologies

Selectivity is achieved due to the availability of several product ions per compound, facilitating cross-comparison of independent concentration measurements from singular product ions. N, N-Dimethylformamide (DMF) was introduced as it is the most likely interferent for NDMA via its 13C isotope peak.
When observed, this interference can be corrected by subtraction from the apparent NDMA signal. This correction was considered in the concentration data presented in this article.
In addition to possible interference by DMF, increased levels of residual solvent can also influence the calculation of concentrations in SIFT-MS due to excessive consumption of reagent ions.
The non-linear responses to drug product mass for H3O+ and O2+ show high concentrations. This phenomenon must be understood and compensated for in method development.
In the study presented here, the NO+ reagent is usually more resilient to this effect than H3O+ and O2+ as it is less sensitive to regularly used solvents such as ethanol or isopropyl alcohol.
Figure 4 shows a full scan analysis of a particular drug product with spectral features arising from these labeled solvents.

Figure 4. SIFT-MS full-scan mass spectra of the two ranitidine-containing drug products containing relatively high levels of residual solvents (a) isopropyl alcohol in Product 1 and (b) ethanol in Product 2. The largest spectral features peaks are from the reagent ions, H3O+ (m/z 19), NO+ (30), and O2 + (32). Image Credit: Syft Technologies
Drug product samples
The study presented in this article focused on method development rather than the quantity of drug samples tested. Only two commercial ranitidine products were assessed for NDMA content via analysis.
Powdered samples (100 to 500 mg) were introduced to 10 mL headspace vials for headspace analysis without ensuing dissolution in water (i.e., of the powder itself) in a method known as “direct analysis.” This allowed a smaller headspace vial to be used compared with the “standard” recommended volume of 20 mL, enhancing sensitivity.9
Results and discussion
This study evaluated the quantitation of NDMA in the gas phase using headspace-SIFT-MS, and NDMA in ranitidine products was quantitatively analyzed.
NDMA detection linearity and limit of quantification
When developing the method, it was first necessary to confirm the linear detection of NDMA and acquire an estimated limit of quantification (LOQ) for headspace analysis.
Figure 5 displays two datasets created by serial dilution of fully evaporated NDMA solutions. The increased range on the left is due to the full evaporation of 1 μL of commercial NDMA standard (5 mg mL-1 in methanol).
A 1 μL aliquot of the headspace was diluted in a nitrogen-filled 20 mL sample vial. Aliquots from this vial were inserted into five vials at 250, 500, 750, 1000, and 1250 μL, corresponding to the NDMA mass displayed on the x-axis.
The lower range shown in Figure 5(b) required pre-dilution of the commercial methanolic standard in 50 % acetone before creating the vapor-phase stock via a 0.5 μL spike into a 20 mL headspace vial. This is the reason for the four-fold dilution and various generated levels.

Figure 5. NDMA response in presence of (a) methanol and (b) 50:50 methanol:acetone mix. See the text for details on how these curves were created. Image Credit: Syft Technologies
The three ions targeted by this NDMA analysis are displayed as they demonstrate the second matrix effect, shown by the presence of solvents (methanol and methanol with acetone).
Acetone was employed as a dilutant to replicate the non-linear response in NO+. This was tried because acetone reacts faster with this reagent ion than methanol, but the NO+ remained steadfast.
While the solvents originate from the calibration mixture rather than a drug product, the effect is identical. Due to a lack of chromatographic separation, the solvents are present in the ionization region of the SIFT-MS instrument (the flow tube) and rival NDMA for the selected SIFT-MS reagent ion.
At the high end of the concentration scale in Figure 5(a), there is about 60 ppmV of methanol in the headspace. Methanol does not affect NO+, but H3O+ and, to a lesser extent, O2+ are increasingly over-reported as concentration rises.
Due to this behavior, the NO+ product ion (m/z 74) is employed as the quantitation ion, while H3O+ and O2+ are labeled as qualifier ions. Figure 6 outlines the combined range for the quantitation ion.

Figure 6. NDMA across full range showing just the quantitation ion, NO+ m/z 74. Image Credit: Syft Technologies
Table 3 outlines the signal-to-noise ratio (S/N), limit of detection, and LOQ for gas-phase SIFT-MS NDMA analysis.
These values are typical of 2.5 mL aliquot of headspace injected at 100 μL s-1 and were quantified using the low-concentration headspace injection shown in Figure 3. This data estimated the LOQ for drug products as about 2 ng g-1 for a 500 mg sample.
Table 3. Signal-to-noise (S/N), limit of detection (LOD), and limit of quantitation (LOQ) for gas-phase SIFT-MS analysis of NDMA in a 2.5-mL aliquot injected at 100 μL s-1. Source: Syft Technologies

Quantitative analysis of NDMA in solid drug product
MHE allows absolute concentrations in condensed phases independent of the matrix to be determined.10 MHE is a powerful method because it does not require introducing complicated matrix-matching procedures for direct quantitative analysis of solid dose forms.
The repeat-analysis methodology of the SIFT-MS technique is significantly cheaper due to rapid sample analysis. However, the technique aims to correlate the first injection with full MHE and apply a correction factor rather than run full MHE on all samples.11
Successful MHE requires analysis across a dynamic range of about two orders of magnitude. As a result, the first step is optimizing the sample quantity needed from static headspace analysis (at equilibrium; see Figure 7). For NO+, signal levels indicated that 500 mg of drug product could be applied.

Figure 7. Measured headspace concentration of NDMA using SIFT-MS as a function of sample mass for two ranitidine drug products. Image Credit: Syft Technologies
Therefore, full MHE was performed, and Figure 8 displays the results recorded over six injections, plotted against the natural logarithm of concentration. The ions in Product 1 (Figure 8[a]) do not correlate linearly with injection number, while NO+ and O2+ behave well for Product 2.

Figure 8. MHE-SIFT-MS results (natural logarithm of headspace concentration as function of injection number) for NDMA in 500-mg samples of two ranitidine drug products. Image Credit: Syft Technologies
The NDMA concentration is at the baseline by the sixth injection for both drug products under the incubation conditions applied. This demonstrates that in cases where it is impossible to employ the conventional extrapolation approach to establishing total concentration in the drug product, individual concentration measurements can still be totaled.12
For Product 2, the traditional area-under-the-curve approach was employed to determine the final concentration. For Product 1, the values recorded for injections 1-6 were summed.
The concentrations of NDMA in products 1 and 2 were 68 and 328 ng g-1, respectively. These values align with the range reported to the US FDA for ranitidine products.13
Full MHE-SIFT-MS provides a sample throughput of 40 samples per day with a 30-minute incubation, in contrast with MHE-gas chromatography (GC) analysis, which can only run five samples per day.14
Despite some non-linear MHE curves, the results presented here are highly repeatable, as indicated by triplicate measurements acquired for 300 mg samples, shown in Figure 9.

Figure 9. Repeatability of triplicate MHE-SIFT-MS measurements of NDMA (using NO+ 74) in 300-mg samples of the two ranitidine drug products. Image Credit: Syft Technologies
This data used the whole six-injection MHE approach with no internal standard applied, as is common practice with SIFT-MS. This demonstrates excellent repeatability, even in the case of non-ideal matrices.
This repeatability feature allows single headspace injection to be used per sample rather than full MHE, as the latter is repeatably related to the initial injection, as demonstrated during the analysis of polystyrene using MHE-SIFT-MS.14
It is important to note that the sample is required to be at equilibrium. Figure 10 shows the correlation between the first injection and the sum of injections (1-6) for the repeatability data presented in Figure 9.

Figure 10. Repeatability of the ratio of first injection to the sum of all injections for the two ranitidine drug products shown in Figure 9. Image Credit: Syft Technologies
The replicates demonstrate excellent repeatability close to 0.6 of the total NDMA in the sample partitioning to the headspace in the initial 30-minute incubation cycle at 60 °C, indicating that upwards of 250 samples can be screened per day for very low nanogram-per-gram quantities of NDMA with headspace-SIFT-MS.
This represents about four times greater throughput for GC and liquid chromatography with automated sample preparation and over six times greater for manual sample preparation.
Conclusions
A high-sensitivity headspace-SIFT-MS analysis can detect NDMA at the extremely low ng g-1 range. Headspace-SIFT-MS provides a greatly improved time to result and larger throughputs than traditional chromatographic methods.
Running quantitative analysis directly from solid/powder from a single incubation cycle is possible through correlation with MHE-SIFT-MS.
The method has demonstrated simple sample preparation (simply adding powder to a vial) and simple operation throughout.
SIFT-MS analysis represents an industry-proven technology ready for use in the QA/QC lab and process line.
References and further reading
- Hera, D., et al. (2017). Negative Reagent Ions for Real Time Detection Using SIFT-MS. Environments, 4(1), p.16. https://doi.org/10.3390/environments4010016.
- Langford, V.S., et al. (2015). Real-time measurements of nitrosamines in air. International Journal of Mass Spectrometry, 377, pp.490–495. https://doi.org/10.1016/j.ijms.2014.04.001.
- Perkins, M. and Langford, V.S. (2021). Application of Routine Analysis Procedures to a Direct Mass Spectrometry Technique: Selected Ion Flow Tube Mass Spectrometry (SIFT-MS). Reviews in separation sciences, 3(1), pp.e21003–e21003. https://doi.org/10.17145/rss.21.003.
- Perkins, M.J. and Langford, V.S. (2021). Standard Validation Protocol for Selected Ion Flow Tube Mass Spectrometry Methods Applied to Direct Headspace Analysis of Aqueous Volatile Organic Compounds. Analytical Chemistry, 93(24), pp.8386–8392. https://doi.org/10.1021/acs.analchem.1c01310.
- Perkins, M. and Langford, V.S. (2022). Multiple Headspace Extraction-Selected Ion Flow Tube Mass Spectrometry (MHE-SIFT-MS). Part 1: A Protocol for Method Development and Transfer to Routine Analysis. Reviews in separation sciences, 4(1), pp.e22001–e22001. https://doi.org/10.17145/rss.22.001.
- Smith, D., McEwan, M.J. and Španěl, P. (2020). Understanding Gas Phase Ion Chemistry Is the Key to Reliable Selected Ion Flow Tube-Mass Spectrometry Analyses. Analytical Chemistry, 92(19), pp.12750–12762. https://doi.org/10.1021/acs.analchem.0c03050.
- Golob, N., et al. (2022). Nitrocellulose blister material as a source of N-nitrosamine contamination of pharmaceutical drug products. International Journal of Pharmaceutics, 618, p.121687. https://doi.org/10.1016/j.ijpharm.2022.121687.
- Boltres, B. (2021). Evaluating Nitrosamines from Elastomers in Pharmaceutical Primary Packaging. PDA Journal of Pharmaceutical Science and Technology, 76(2), pp.136–150. https://doi.org/10.5731/pdajpst.2021.012645.
- EMA (2021) Questions and answers for marketing authorisation holders/applicants on the CHMP Opinion for the Article 5(3) of Regulation (EC) No 726/2004 referral on nitrosamine impurities in human medicinal products, EMA/409815/2020. Available at: https://www.ema.europa.eu/en (Accessed: 15 September 2022).
- US FDA (2019) Laboratory Tests | Ranitidine. Available at: https://www.fda.gov/drugs/drug-safety-and-availability/laboratory-testsranitidine (Accessed: 28 September 2022).
- US FDA (2021) Guidance for Industry. Control of Nitrosamine Impurities in Human Drugs. Available at: https://www.fda.gov/media/141720/download (Accessed: 15 September 2022).
About Syft Technologies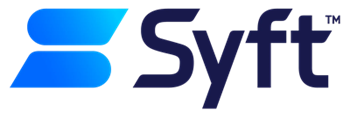
Syft Technologies is the world leader in real-time, direct injection mass spectrometry with more than 20 years of SIFT-MS expertise. The unique attributes of Selected Ion Flow Tube Mass Spectrometry (SIFT-MS) have enabled our customers to remove bottlenecks and solve daunting analytical challenges. The results are improved capacity and organizational efficiency for our customers and a safer world.
Syft Technologies supports a broad range of worldwide industries including semiconductor manufacturing, pharma and CDMOs, environmental protection, automotive, food, flavor and fragrance, and many more. Syft has offices throughout the world offering 24/7 service and support including those in New Zealand, Korea, Taiwan, Singapore, Germany and the U.S.
SIFT-MS delivers real-time, chromatography-free direct analysis of compounds that traditionally require intensive sample preparation. No sample preparation is required for even complex matrices and high-humidity samples. SIFT-MS analyzes compounds that cannot be easily targeted by traditional chromatographic methods such as formaldehyde, hydrogen sulfide, ammonia, ethylene oxide, and nitrosamines. SIFT-MS is industry-proven, providing non-technical operators with the laboratory-grade chemical analysis presented in a format that they can easily understand and act on. Syft’s flagship product is the Syft Tracer which delivers routine and repeatable analysis of volatile compounds.
Sponsored Content Policy: News-Medical.net publishes articles and related content that may be derived from sources where we have existing commercial relationships, provided such content adds value to the core editorial ethos of News-Medical.Net which is to educate and inform site visitors interested in medical research, science, medical devices and treatments.