An interview with Dr. Colin Sanctuary, CEO, QGel, conducted by April Cashin-Garbutt, MA (Cantab)
What are organoids and what impact could they have on biomedical science and personalized medicine?
Organoids are bits of tissue grown in vitro, in a near-physiological 3D setting. The particularity consists in the fact that organoids retain features of the patient they are derived from, even when cultured outside of the human body. This is very different from what traditional, conventional cell culture has been able to do over the past 50 years or so.
The ability to culture patient copies of certain organs, whether healthy or diseased, provides an opportunity for drug developers and biomedical science in general to have a more relevant system and a more predictive model for finding out which drugs work better for which indication and at which dosage.
In what ways does this approach differ from traditional cell culture?
There are two main differences. The first is that we're taking patient-derived cells, which is very important, because when you take a sample from a patient, you don’t have just a single cell type; you have a whole agglomeration of different cells in that tissue sample that need to be isolated, dissociated and grown in an in vitro system. This is complex to do.
With traditional systems the cell lines are immortalized; which means they are originally derived from humans, but have been isolated, passaged and simplified to the point that you're getting only a limited representative picture of a certain feature of a certain cell type. Hence, it does not represent the heterogeneity or the complexity of what actually happens in the patient.
Having an oversimplistic system with conventional cells is sometimes advantageous and we cannot write it off or say it is not useful, but we can say that it has its limitations.
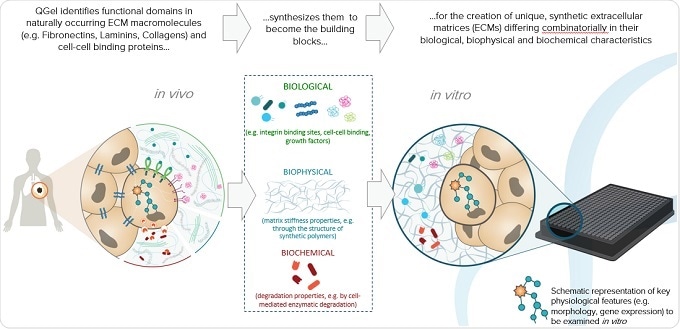
So far, what has limited the progress of organoids in drug discovery and diagnostics?
Progress is mostly limited by trying to find the right environment or media conditions we need to provide for the patient cells to divide, form, grow and develop into organoids. It’s about how to identify those components which recreate the physiological microenvironment.
We have a number of experts in biology who've identified these media systems, liquid formulations that when combined with a matrix enablespatient derived-cells to differentiate and turn into an organoid. That's the biological aspect, which, today, is fundamental research and usually does not require large-scale studies. For such studies, you’re talking about a small lab that can treat or test perhaps five or ten patients and acquire meaningful biological results
Drug discovery and diagnostics both industrial applications present a different set of challenges because the quality of the biological result will require high quality material in quantities to enable sufficient throughput. . So in other words, the limitation relates to the inability to scale organoid applications to be able to adequately do drug discovery or personalized diagnostics.
So what are these critical materials? This is where two new factors come into play when we talk about making these applications a reality. The first one is cell source. Taking cells from a patient and putting them into culture at the bedside or at the drug discovery facility is a challenge. It's one of the problems that arises when you have a limited, rather than an “unlimited” supply of immortalized cell lines.
Identifying and solving the problems of sourcing primary cells is one aspect. The other very important aspect is that organoid research papers that have been published in the high-impact journals are all based on animal-derived 3D matrices, or basement membrane laminin-rich gels.
The very well-known problem with these types of animal gels, is that they are not scalable namely due to its batch to batch variability. They work for fundamental biological research, but until now, no gel system provides the ability to go from o dozens of tests to thousands or even hundreds of thousands of tests, without compromising on scientific results.
There has been tremendous progress with organoids on the fundamentalbiology level, which has been published in a wide range of high impact journals. Recently, we were at the AACR in Washington DC and witnessed that organoids are spoken about everywhere among academics. However, if you walk across to the building next door where the industrial presentations are held, very few spoke about organoids, but rather about more conventional methodologies. From what we saw, this is because they have not found a cell source and cannot even consider scaling up the applications because of these non-scalable animal-derived matrices.
How important is the 3D environment when growing cells?
Depending on the cell type, growing cells can be very easy or extremely complex. For certain applications, growing cell lines in a 2D plate is largely insufficient. After speaking with drug developers and academics, we can see that 2D cell culture is widely used and we believe that it's here to stay for quite some time. Now, that's for the simple cells; those cells that are easy to grow, have known features and known cell media growth conditions and so on.
Then, there are more complex cells, the patient-derived cells, which can be extremely difficult to grow. Here, 2D just does not work. 2D technologies are largely insufficient to reproduce the complex physiology of the human body and another system is needed. What has been shown to work is 3D matrix-based systems – taking complex cell types that grow in a 3D environment that retain and express certain behaviors.
In some cases, 3D is not needed, but in very complex cases or when the system needs to predict clinical results, 3D is a requirement.
Can you please outline how QGel have developed a near-physiological 3D matrix?
Our approach has been very different from what is usually done. I think most people start with biology and say "Let's take a cell system that can't be successfully cultured and try different technologies to make it grow.” Then, out of the 20 technologies that these people have tried, the one seemed to work best turns out to be incompatible with applications in drug discovery and diagnostics
We've taken another approach where we say "Okay, forget the cell type. Let's take a look at what's surrounding the cell. Because the environment around the cell plays such a tremendous role. That’s where we start from."
This is a very well published field, at least in the biomaterials world - so not really biology, but rather biomaterials inspired by nature, that consist of different molecules and different biophysical forces such as the stiffness of the gel surrounding the cell.
A conceptual example I can give that everyone can relate to is eye tissue. The matrix surrounding the cells of the eye is very soft, whereas with bone, , the surrounding matrix is very stiff. Cartilage would be somewhere between the bone and the eye tissue.
These are the type of environmental factors that we were looking to nature for, as inspiration. First we would define a soft or hard matrix, based on what type of organ we are looking at. Then again, biomechanical engineers have performed serious analyses of the difference in stiffness between the environments of different organs.
Stiffness is only one aspect and then there's the biological aspect. There are some growth factors that are required for the kidney to grow, for example, whereas those same growth factors would not make brain tissue grow. The cocktail of growth factors required for one organ is not the same as that for another organ. This is also very well published. We define what specific cell type is needed and then we incorporate the relevant factors into a gel with a certain stiffness.
Finally, there is the biochemical aspect. We are constantly remodeling ourselves. Take bones for example: there are cells that eat up bone and cells that create bone, with the whole human skeleton being replaced every 12 months. A key step in this replacement is to degrade the matrix, which is a biochemical process. We look at this and many other processes to be inspired and define how to incorporate biochemistry into our gels. It's a very complex technology which took nearly 20 years of R&D development to get to where we are today.
We've combined all these factors into a highly complex technology: stiffness, biological growth factors and biochemical degradation factors, and incorporated them into a product that just works. Each formulation we create is unique and specific to each cell type being researched.
Today, you send a brain gel to a client that uses brain cells and, so long as they follow the right media conditions and so on, they're guaranteed to reproducibly grow the brain cells. We could provide another type of gel for heart tissue, for example, and they'd be able to grow the heart cells in a straightforward manner.
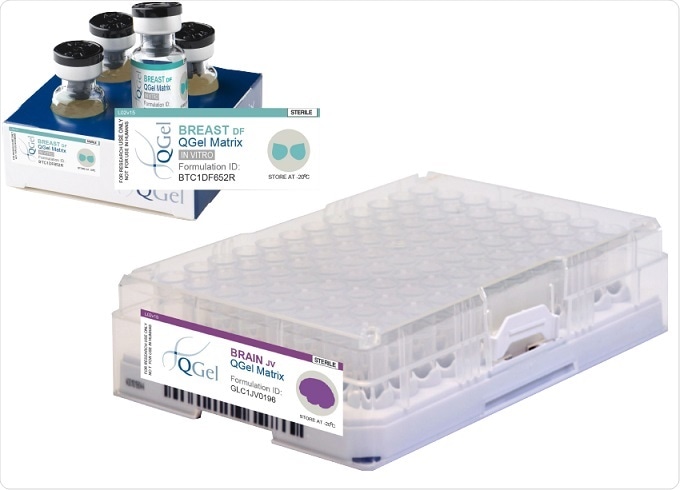
How can the technology be adapted to different cell types?
We’ve built a library of over a hundred gel types and when combined with various media conditions, QGel is able to approximate the different matrices within the body. We've miniaturized it; we work with robots that handle very small volumes and we have a scalable solution.
As we have done that, a client or a research institute could say to us "We have a cell type that we can't get to grow and we would like to find a near-physiological 3D matrix that allows it to grow." We can take those cell types, or they could do it at their facility, and we perform an initial screen of several dozen gels but can go up to a hundred different gels if necessary. Then, we get a readout that tells us which gel type best promotes growth of those specific cells.
This is how we've developed and fine-tuned our organoid gels, and now we are even going further with projects that aim to move the use of organoids into the clinic and into drug development pipelines.
Will it be possible to manufacture the 3D matrix on a large scale for drug screening and other applications?
It will, with the QGel 3D Matrix. The company was founded on the idea that one day the biology would work, but for the past four or five years we have been focused on the upscalability of this technology.
In 2008-2009, the gel had only been manufactured on a microgram level and it would cost several thousand dollars per application, which would just not be feasible for the general scientist. However, now we can manufacture on the kilogram level and costs have been reduced significantly so that we're comparable in price to the standard gel matrices that are derived from animals.
This is a big step forward. Manufacturing is only one side of the story for upscaling. The other side, which is extremely important, is related to workflow and usability. If you had a product consisting of 20 different components that needed to be assembled by the customer at the time of drug screening or diagnostics, then you could forget it. You need to be able to deliver a product that can be shipped at room temperature and has a shelf life that means it doesn’t need to be used within a day of receiving it.
These are practical, non-scientific questions, but these factors will make it or break it when it comes to using the product. We've worked for three years adapting our gel and working on the distribution quality parameters of our product and worked closely with a number of pharmaceutical laboratories equipped with high-throughput automation equipment, to make sure that the products we deliver are compatible.
I can say with confidence, as this has been the direct feedback from our clients, that our solution is best in class for this type of drug discovery and upscaled 3D solutions with gels.
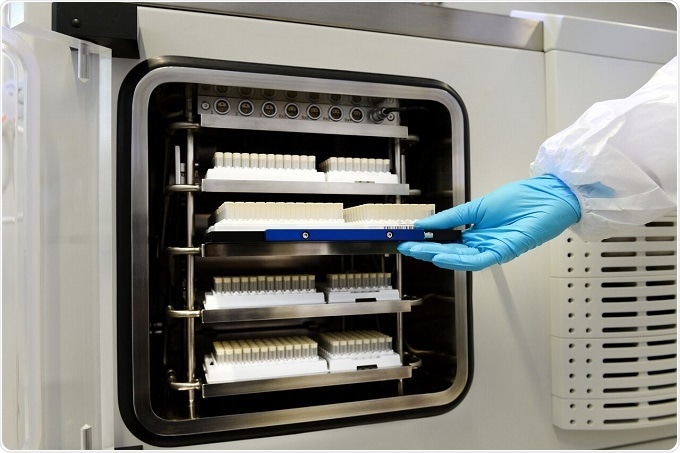
What impact do you hope QGel’s technology will have on drug discovery?
With more predictive models, cell based preclinical studies have better chances to be successful. We hopet that scientists will be able to focus more resources on promising drugs, and discontinue unsuccessful drug campaigns earlier. There are two branches to this. The first one is drug discovery and the other is personalized medicine.
If you have a more predictive model with a biological system that represents a patient or a pool of patients, you will be able to better predict and increase the success rate of a compound as it moves down the drug development pipeline.
Currently, if 20 compounds make it into clinical studies, only one will make it to approval. The cost of failure, is tremendous and this is well documented. If we can reduce that rate and make it so that five of 20 going into the pipeline reach the market, then the pharmaceutical industry will have five new products on the market. That could potentially recoup their cost of development five-fold. This is how we can value where QGel could go with this drug discovery application.
In terms of applications in personalized medicine, being more predictive when it comes to patients means improving care, and eventually saving lives. If a patient is sick with cancer, for example, and we're able to take a biopsy of that cancer and grow it into organoids or tumoroids in a scalable manner, then a panel of approved drugs can be tested on the patient’s cancer itself, without it being necessary to test the patient directly. The clinician could then get a readout of which drug, drug combination, andat which dosage, that patient should receive.
What do you think the future holds for growing organoids and personalized medicine?
On the scientific, fundamental level, I think the growth of organoids is a booming field. When you look at citations and how often an organoid is used, you see there’s been a revival since 2009. I think we're still in the early days of organoid technology when it comes to patient-relevant applications, with organoid models being used by pharmaceutical companies.
I predict that, once the first clinical studies are performed, and the first results are successful, there will be a shift in how people are treated. It will change drastically.
The guesswork that clinicians are forced to do when choosing the right therapy for the patient will not be eliminated, but it will be very much reduced. From a health economics point of view, this will equate to tremendous savings on healthcare costs for countries or insurance companies, depending on the system.
For example, the NHS has put initiatives forward to reduce the cost of healthcare tremendously, with personalized- or precision-medicine approaches. However, other than genomics, the precision medicine approaches are not bedside yet. They don't take into account the day the patient is sick. You can use genomic analysis to predict the probability that someone will get cancer, but that doesn't help very much. When a person does get cancer is when the stopwatch starts on whether they’re going to survive or not, which is when we need tools to better predict treatment strategies for that patient.
I think the future is extremely bright, but there are still some critical challenges to be overcome, before we can talk about organoids as a solution for drug discovery and personalized medicine.
What is QGel’s vision?
QGel's vision is to be a key player in enabling technology to allow more predictive models that could have immediate application today in drug discovery, but it goes hand-in-hand with personalized medicine.
I envisage QGel being a critical provider, not only in materials, but also in the know-how on how to provide access to organoid technology where it matters, which is in the pharmaceutical industry where companies are trying to develop drugs that can save patients’ lives.
On the other hand, we would transfer this expertise and know-how in a simple way that can be scaled and adopted in a hospital setting or a chemistry center setting, where organoids could also be used to save patients’ lives. This is our vision and it's really quite exciting right now, given the results we're getting.
What main challenges still lie ahead?
The groups focused on personalized medicine today are fundamental researchers who have access to patient cells that are close to the hospital, on a very small scale. I think if you count the number of organoid experts, you could probably fill a small room.
However, from the clinical viewpoint, even when you look at just the developed world, clinicians face the challenge of addressing what is a cancer epidemic. If you look at Europe and the US, there are millions of new cancer cases every year.
Trying to turn an organoid technology that works for a handful of people into something that clinicians take seriously and use every day is going to be a significant challenge. Going back to QGel's vision, at some point clinicians must buy into this technology and, in general, clinicians are so busy treating the patients they see and have become emotionally attached to, that it's going to be a big challenge to bring what's happening on the fundamental side over to the believers and the first movers on the clinical side.
Where can readers find more information?
QGel
About Dr. Colin Sanctuary
Chief Executive Officer, Board Member & Founder
Colin is a Biomedical Engineer and he held positions at Straumann and Medtronic before founding QGel in 2009.