The ability to contain the coronavirus 2019 (COVID-19) pandemic is limited in the ability to detect at the right time, monitor effectively, and target to cure. However, severe acute respiratory syndrome virus coronavirus 2 (SARS-CoV-2), the causative agent of COVID-19, is far from under control. So far, it has infected over 55.57 million individuals and claimed the lives of over 1.33 million globally. Many people are symptom-free but infective carriers - this dangerously augments the transmission of the virus.
Current detection methods involve: 1) RT-PCR (Reverse Transcription Polymerase Chain Reaction), where the results take about a day or more due to complex sample processing in specialized, well-instrumented laboratories, and 2) Immunoassays, that can detect viral loads above 50 million copies/mL (useful for the isolation of highly infectious individuals). The lack of sensitivity and robustness against sample processing and reaction times delays isolating infected carriers - severely hampering the suppression of infection chains.
A recent study presents a bioelectronic sensor platform to detect protein at the single-molecule level that is simple, rapid, and robust enough for widespread POC (point-of-care) or bedside application. The research published as a preprint on the medRxiv* server introduces a modular nanobody-organic electrochemical transistor sensor that provides results after a 10 minutes exposure to 5 µL of unprocessed samples, maintains high specificity and single-molecule sensitivity in human saliva or serum.
Instead of the classic antibodies (or antibody fragments), nanobodies are used here. Nanobodies are compact recognition modules made from the antigen-binding domain (VHH) of an unusual class of heavy chain-only antibodies found in Camelids. The sensor used in this study combines a new solution-processable organic semiconductor material in the transistor channel with the high-density and orientation-controlled bioconjugation of nanobody fusion proteins on disposable gate electrodes.
This study is claimed to be the first where nanobodies are combined with OECT (organic electrochemical transistors) technology. Key features in this process are (i) the use of a novel organic semiconductor that allowed for the first practical implementation of an accumulation-mode OECT and (ii) the controlled biofunctionalization of the sensor surface with (iii) custom-engineered nanobodies.
In this study, the researchers from King Abdullah University of Science and Technology (KAUST), King Faisal Specialist Hospital & Research Center, KAUST Solar Center (KSC), Saudi Arabia, the University of Oxford, Imperial College London, United Kingdom, and Université de Montpellier, France, tested for three different target proteins. To optimize multiple parameters of the sensor, they used the anti-GFP nanobody-conjugated gate electrode as a model. The sensor responds within a dynamic range of six orders of magnitude. This sensitivity is on-par with commercial colorimetric ELISA assays, which, however, take hours to complete, require larger sample volumes, and have a far narrower dynamic range.
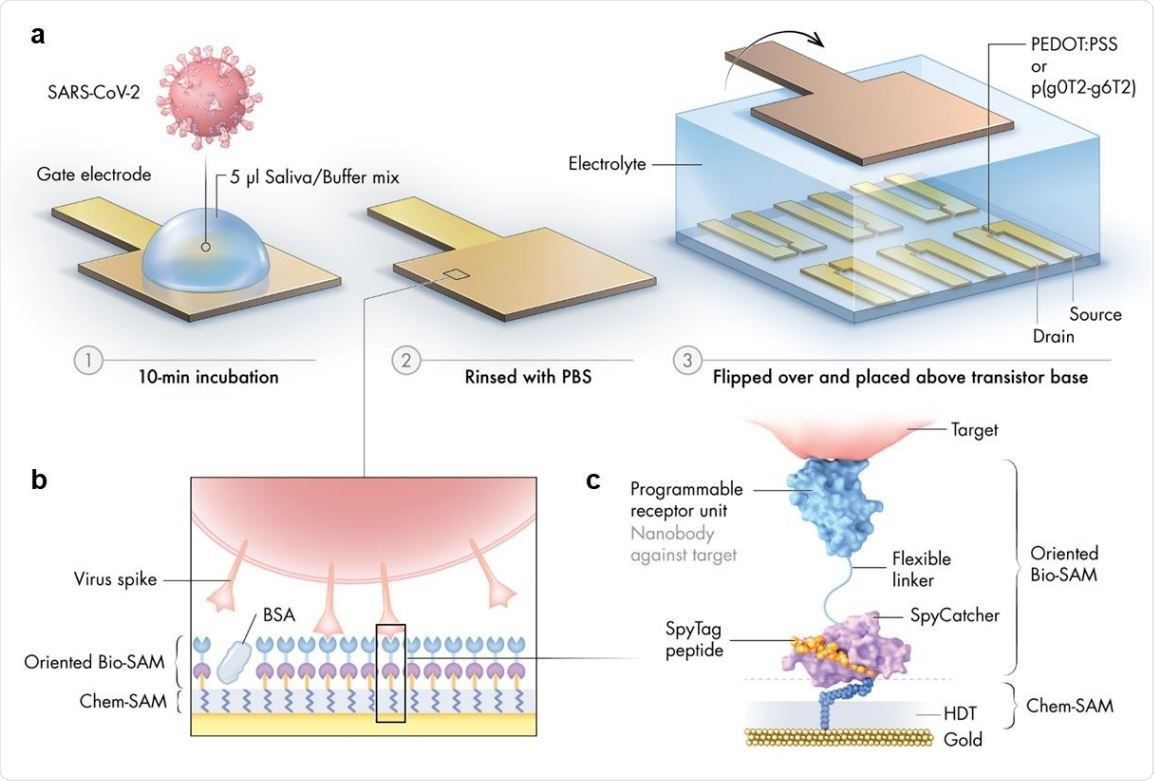
Schematic illustration of the nanobody-functionalized OECT sensor. a) Operation: The gate electrode is exposed to a mix of sample (such as saliva) and binding buffer (1), rinsed with PBS (2), and mounted on top of the OECT channel for signal acquisition (3). b) Gate functionalization layers. Chemical and biological monolayers (Chem-SAM and Bio-SAM) are self-assembled on the gate electrode surface. c) Molecular architecture. A synthetic SpyTag peptide is chemically coupled to the 1,6-hexanedithiol (HDT) monolayer to form a Chem-SAM. The nanobody-SpyCatcher fusion protein then attaches itself to this chemical layer through the autocatalytic formation of a covalent SpyCatcher-SpyTag bond, forming the Bio-SAM. The nanobody domain defines sensor specificity and is interchangeable. Bovine serum albumin (BSA) is physisorbed on the sensor surface during the final step of functionalization to prevent nonspecific binding.

This news article was a review of a preliminary scientific report that had not undergone peer-review at the time of publication. Since its initial publication, the scientific report has now been peer reviewed and accepted for publication in a Scientific Journal. Links to the preliminary and peer-reviewed reports are available in the Sources section at the bottom of this article. View Sources
Thus, the researchers show that this platform is broadly applicable and is only limited by the availability of antigen-specific nanobodies. Usually, the currently available organic mixed (semi)conductors are not stable; the researchers demonstrate that this sensor has exceptional operational and environmental stability.
The researchers find that observation of binding at such low concentrations is due to the high-density nanobody-functionalized gate electrode that operates as a kinetic trap for target molecules. The kinetic stability of the complex (koff) (~1.5 × 10-4 s-1 corresponding to 80 min half-life) and the diffusion rate dictate such high sensitivity observed in this study.
The sensor is reusable, easy to manufacture, and highly modular. It can detect specific single protein molecules reliably on millimeter-sized disposable electrodes in uncontrolled, ambient environments. This sensor is also quantitative and distinguishes concentrations over 8 to 10 orders of magnitude (attomolar to nanomolar).
Design and characterization of the biofunctionalized gold electrode. a) Annotated sequence of the recombinant fusion protein combining a nanobody recognition module (blue) with the SpyCatcher domain (brown) with an intervening flexible linker (short-dashed line). b) Structural model of the complete biorecognition layer assembled from HDT and SpyTag (Chem-SAM) and nanobody-SpyCatcher fusion protein (Bio-SAM) (based on PDB structures 4MLI and 3OBO). Unstructured, flexible linker regions are indicated by short-dashed lines drawn to scale. c) High resolution S 2p XPS spectra of the gold electrode recorded after immobilization of HDT, SpyTag peptide, and the nanobody-SpyCatcher protein using final conditions with BSA added to the immobilization. d) QCM-D profile measuring the coupling of SpyTag peptide and nanobody-SpyCatcher fusion protein (without BSA) in real-time, starting from an HDT-coated gold electrode.
The interdisciplinary team demonstrates that the platform's functioning is fast; from sample to test results, it takes less than 15 min. It does not require sample preparation; unprocessed saliva or serum samples can be directly used.
The researchers confirmed single-molecule sensitivity and high specificity of SARS-CoV-1 or MERS nanobody-functionalized OECTs in buffer samples and saliva in detecting SARS-CoV-2 or MERS-CoV. They have further validated the sensor's performance with the clinical unprocessed nasopharyngeal swab and saliva samples from COVID-19 patients and healthy volunteers and demonstrated a sensitivity comparable to RT-PCR methods. RT-PCR often detects membrane-associated RNA long after the infection has cleared from the body.
In conclusion, the researchers have bioengineered a nanobody-functionalized OECT sensor, introducing a label-free electrochemical immunosensing platform with single protein molecule sensitivity and a broad dynamic range. The sensor can also be rapidly adapted to any target for which nanobodies exist or can be raised.
"The speed, performance and versatility of our nanobody functionalized OECT, and its compatibility with many sample types, suggest that this platform can complement or replace a wide range of clinical and non-clinical diagnostic assays," the researchers write.
The COVID-19 crisis highlights a critical limitation of our current POC diagnostics toolbox. This platform introduced here could not have come sooner.

This news article was a review of a preliminary scientific report that had not undergone peer-review at the time of publication. Since its initial publication, the scientific report has now been peer reviewed and accepted for publication in a Scientific Journal. Links to the preliminary and peer-reviewed reports are available in the Sources section at the bottom of this article. View Sources
Journal references:
- Preliminary scientific report.
A nanobody-functionalized organic electrochemical transistor for the rapid detection of SARS-CoV-2 and MERS antigens at the physical limit, Keying Guo, Shofarul Wustoni, Anil Koklu, Escarlet Díaz-Galicia, Maximilian Moser, Adel Hama, Ahmed A. Alqahtani, Adeel Nazir Ahmad, Fatimah Saeed Alhamlan, Iain McCulloch, Stefan T. Arold, Raik Grünberg, Sahika Inal, medRxiv 2020.11.12.20228874; Doi: https://doi.org/10.1101/2020.11.12.20228874, https://www.medrxiv.org/content/10.1101/2020.11.12.20228874v1
- Peer reviewed and published scientific report.
Guo, Keying, Shofarul Wustoni, Anil Koklu, Escarlet Díaz-Galicia, Maximilian Moser, Adel Hama, Ahmed A. Alqahtani, et al. 2021. “Rapid Single-Molecule Detection of COVID-19 and MERS Antigens via Nanobody-Functionalized Organic Electrochemical Transistors.” Nature Biomedical Engineering 5 (7): 666–77. https://doi.org/10.1038/s41551-021-00734-9. https://www.nature.com/articles/s41551-021-00734-9.
Article Revisions
- Mar 30 2023 - The preprint preliminary research paper that this article was based upon was accepted for publication in a peer-reviewed Scientific Journal. This article was edited accordingly to include a link to the final peer-reviewed paper, now shown in the sources section.