The coronavirus disease 2019 (COVID-19) pandemic has highlighted the need for new broad-range antiviral medications. While many bacterial infections can be treated with the same medications, antiviral treatments are often much more specific and, in many cases, there is not an agent that targets the pathogen responsible for the disease.
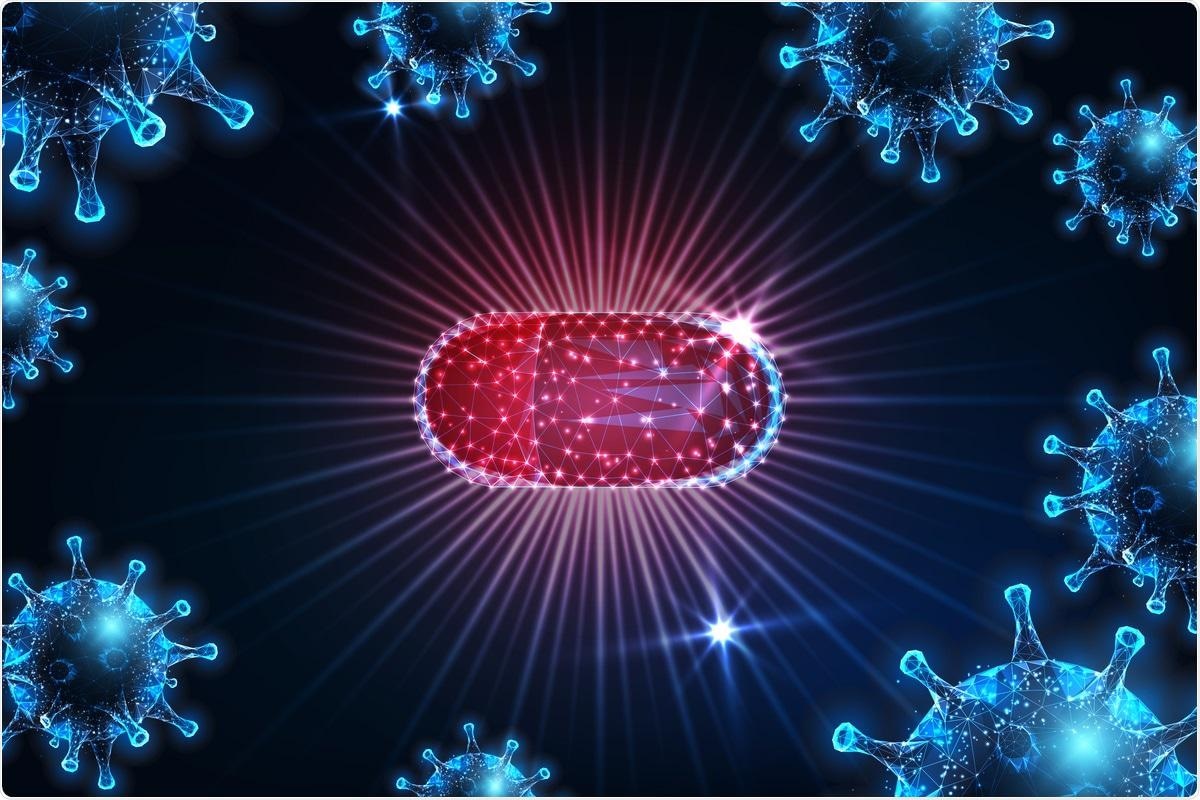
Study: A natural broad-spectrum inhibitor of enveloped virus entry, effective against SARS-CoV-2 and Influenza A Virus in preclinical animal models. Image Credit: Inkoly / Shutterstock.com

*Important notice: bioRxiv publishes preliminary scientific reports that are not peer-reviewed and, therefore, should not be regarded as conclusive, guide clinical practice/health-related behavior, or treated as established information.
The lack of treatments that are directed against the severe acute respiratory syndrome coronavirus 2 (SARS-CoV-2) has forced healthcare workers to treat infected patients with supplementary oxygen and invasive mechanical ventilation in the hopes that their immune system could eventually fight the infection. This has led researchers to recently investigate the potential of picolinic acid (PA) as an anti-SARS-CoV-2 treatment option.
In vitro testing of PA
In the current study published on the bioRxiv* preprint server, researchers initially theorized that as the endocytic process can be co-opted for viral cell entry, PA could interfere with SARS-CoV-2 infection, as it can interfere with endosome maturation. To this end, the in vitro antiviral efficacy of PA against the H1N1 influenza A virus (IAV) was initially tested at different doses in Madin-darby canine kidney (MDCK) cells.
A dose-dependent reduction in infectious virus counts was observed 48 hours post-infection with IAV, with the most inhibition reported at 2 micromolar (mM) PA. Further exploration with the ‘Swine Flu’ strain of IAV revealed consistent inhibition of viral replication, which led the scientists to further explore the ability of PA to inhibit other important human viral pathogens.
Subsequently, PA was used to treat HEK293T-ACE2 cells, which exogenously express the angiotensin-converting enzyme 2 (ACE2) receptor, following infection with SARS-CoV-2. To this end, PA was found to be effective, with a two-log reduction in viral ribonucleic acid (RNA) in the presence of 2 mM PA, which was a non-toxic dose for the cells.
Further examination with different SARS-CoV-2 variants including the Alpha, Beta, and Delta variants showed consistent results. Furthermore, an investigation of flavivirus infection in A549 cells also showed significant viral inhibition.
In vivo efficacy of PA
Initial toxicity studies in BALB/c mice showed that PA was non-toxic at 20 mg/kg through both intraperitoneal (IP) and oral administration. At 100 mg/kg, PA was non-toxic orally; however, the IP administration of this dose resulted in an approximate 10% loss of body weight.

Picolinic Acid mitigates SARS-CoV-2 replication and pathogenesis in vivo (A) Schematic for PA treatment in hamsters using prophylactic and therapeutic regimens is shown. Prophylactic treatment of hamsters involved administration of PA at 1,2 and 3 days prior to infection, followed by virus challenge at day 0. The therapeutic treatment used dosage during 1,2 and 3 dpi. In both cases, animals were sacrificed 4dpi. (B-D) Results for the administration of PA via IP route showing (B) lung vRNA copy number, total lung weight, and (D) bodyweight of animals up to 4 dpi. (E-G) Corresponding data for oral administration of PA showing (E) lung viral RNA copy number, (F) total lung weight, and (G) bodyweight of animals. qRT PCR and lung weight data shown are from 1 experiment (n=3 per group). Bodyweight data are from 1 experiment and presented as the mean percentage of bodyweight measured at Day 0, n= 2-5 per group. In all cases, One-way ANOVA with Dunnett’s multiple comparisons was performed.*p < 0.05; **p < 0.01. (H) Histology images for all treatment groups including mock-infected and healthy controls. (I) Clinical scoring was based on the following criteria and labeled within inset images as (1) alveolar edema, (2) vascular and perivascular infiltration and (3) alveolar thickening and infiltration. Black arrows indicate vascular infiltration, arrowheads show perivascular infiltration, green arrow show alveolar edema. Scoring was done based on the severity on a scale of 1-4 (1-mild, 2-moderate, 3-severe, 4-very severe). An overall score was given by accumulating the total scores for each criterion.
BLAB/c mice were then challenged with 50 plaque-forming units (PFU) of PR8 IAV. These mice were either treated prophylactically or following infection with PA through both oral and IP routes.
Almost every mouse that received PA treatment showed 100% survival rates, with the exception of the IP prophylactic group, which showed 80%. This was comparable to the control treatment group, which exhibited 20% survival rates. All PA treatments prevented significant weight loss, reduced infectious viral count in the lungs, and reduced pathological features of the disease.
The same experiment was then performed against SARS-CoV-2 using the Syrian Golden hamster model. The IP administration of 20 mg/kg PA reduced lung viral RNA load on day three by about 3- and 1-fold for prophylactic and therapeutic treatment, respectively. Both treatment routes also significantly reduced lung inflammation and body weight loss.
The anti-viral mechanism of PA
Addition assays using SARS-CoV-2 and IAV were used as test viruses to identify the steps of inhibition during a single cycle of virus infection. To this end, pre-treatment of HEK cells with 2 mM PA for three hours before infection mitigated viral infection by 80%, with similar effects observed even after a one-hour incubation.
However, if the PA was added at the time of infection, the effect was much more limited. A similar effect was observed when the experiment was conducted on Vero E6 cells. Further time-of-addition experiments, alongside membrane fusion investigations, revealed that PA acts against IAV by inhibiting the fusion of viral and endosomal membranes.

Picolinic Acid inhibits IAV entry by interfering with Viral-Cellular endocytic membrane fusion and affecting viral membrane integrity. (A-C) A549 cells were either pre-treated for 3hr with 2mM PA, infected with 2 MOI PR8 WT in presence of the drug, and collected 3hr later (−3hr) or the virus was incubated with 2mM PA for 1hr and used for infection (1hr virus + PA). Microscopy data for A549 cells labeled with influenza virus nucleocapsid protein are shown in (A), quantification of NP positive cells is shown in (B), and (C) shows corresponding western blot data. (D-F) A549 cells were first infected with 2 MOI PR8 WT, PA treatment was done 6hr p.i (T0+6hr) and cells were collected a further 3hr later. Microscopy images with quantification and western blot analysis are shown in (D), (E), and (F). ***p < 0.001; ns - not significant using two-tailed unpaired t-test or one-way ANOVA with Dunnett’s multiple comparison test where applicable. Error bars represent mean ± standard deviation. (G) Pictorial representation of virus-endosome membrane fusion assay using R-18 labeled IAV particles. After infection of MDCK cells, the labeled virus particles enter endosomes, and upon pH-dependent fusion of virus and endosomal membranes, results in the de-quenching of R-18 probe dimers and subsequent increase in fluorescence intensity. Created with Biorender. (H) A549 cells were pre-treated with either 2mM PA, 10µM NH4Cl, or 10µM CQ, infected with R18 labeled PR8 WT virus on ice, transferred to a plate reader at 37°C and fluorescence intensity measurements were acquired at 10 min intervals. ***p < 0.001, using one-way ANOVA with Dunnett’s multiple comparison at 90 min time point. (I) HEK293T cells were transfected with plasmids expressing influenza virus PA, PB1, PB2, NP, and NP-firefly luc, along with pRLTK. 2mM PA was added 3hr post-transfection and cells were harvested 8 and 12hr later. Results show luciferase units normalized to untreated control. ns - not significant using two-tailed unpaired t-test. Error bars represent mean ± standard deviation. (J) Concentrated PR8 WT virus particles were incubated with vehicle control or 2mM PA for 3hr, mounted on copper grids, and processed for TEM imaging. Arrows indicate differences in the integrity of viral double-layered membranes in control and treated conditions.
Conclusions
PA has effective wide-range antiviral activity and can effectively treat viral infections in mice models at non-toxic doses for both H1N1 and SARS-CoV-2. Further research and clinical trials are still needed before any final conclusions can be made on the efficacy of PA as an anti-viral agent against H1N1 and SARS-CoV-2.

*Important notice: bioRxiv publishes preliminary scientific reports that are not peer-reviewed and, therefore, should not be regarded as conclusive, guide clinical practice/health-related behavior, or treated as established information.