In a recent study on the medRxiv* preprint server, researchers explored the global surveillance of new severe acute respiratory syndrome coronavirus 2 (SARS-CoV-2) variants.
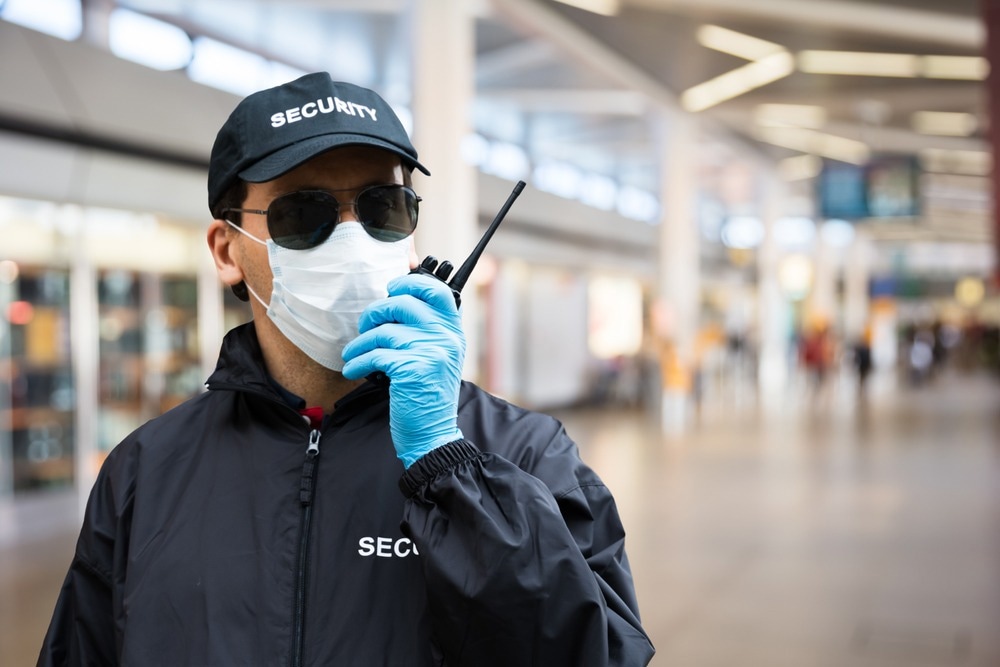
Study: Global surveillance of novel SARS-CoV-2 variants. Image Credit: Andrey_Popov/Shutterstock.com

*Important notice: medRxiv publishes preliminary scientific reports that are not peer-reviewed and, therefore, should not be regarded as conclusive, guide clinical practice/health-related behavior, or treated as established information.
Background
The ongoing pandemic is fueled by new SARS-CoV-2 variants, which are causing illness and death and have the potential to cause societal disruption.
Early implementation of government policies to curb the impacts proved to be more effective but can be costly and should be applied only if necessary. Determining an appropriate response to an emergent variant threat necessitates gathering evidence over time.
However, some countries lack the ability and resources to carry out national surveillance in a timely manner, leading to gaps in global monitoring. The UK adopted large-scale hospital surveillance, but research suggests that detecting new variants by testing UK arrivals would be more efficient.
About the study
In the present study, researchers produced simulations to model the emergence and importation of new SARS-CoV-2 variants with varying infection hospitalization rates (IHR) to the United Kingdom. The study compared the time taken to detect variants through hospital admission, UK arrivals, and the general community.
An epidemic curve that started in a region with a population of 60 million resulted in a single wave. On day zero, the index case was reported. The initial two generations' offspring distribution was fixed at two to establish the epidemic.
The epidemic was assumed to have spread for 16 weeks before the average offspring distribution was decreased to account for control measures and a decrease in susceptible individuals in the population.
Between the 17 and 26 generations, the mean distribution decreased by 0.1 for each successive generation, resulting in a reproduction number of one in the 26th generation. The average of the offspring distribution decreased by 0.01786 from the 27th generation.
The daily prevalence was calculated by adding the simulated cases for each day following the index case's occurrence. The epidemic curve was limited to 300 days in the simulation. A binomial distribution determined the number of incoming travelers incubating or infectious daily.
The daily number of travelers was fixed at 250, and the probability was based on the prevalence of the infection in the origin area on that day. This assumed that infected individuals were as likely to travel as non-infected individuals. A representative sample of travelers, ranging from 10% to 50%, was selected for testing at the border, given that the simulations included at least one infected traveler.
Community cohort surveillance sizes ranging from 20,000 to 200,000 were utilized to detect a simulated infection case within a community setting. Subjects in the surveillance were tested every two weeks. The study involved running simulations on 1,000 destination country epidemics.
The simulations used a binomial distribution to determine the number of daily detections based on daily tests and the simulated prevalence. The test's sensitivity and the percentage of positive tests sequenced were also considered.
Results
The study simulated the time required to detect an imported novel variant by varying the sampling fractions of traveler arrivals in England. The prevalence of infection within the passenger population was assumed to be equivalent to the epidemic curve noted for the origin country over time.
Furthermore, the study found a non-linear correlation between the increase in sampling fraction and the decrease in days to detection. This relationship was observed from 131 days till detection via sampling of 10% of passenger arrivals.
The most significant decline in time to detection occurred when sampling fractions were between 10% to 20%, resulting in a median reduction of eight days. The time gained decreased as the sampling fraction increased.
The study simulated the detection time by testing 50% of hospital presentations in England. The team assumed that the incidence growth in England was equivalent to that in the origin country. Simulations were conducted for scenarios with variant IHRs of 1.0%, 1.5%, 2.0%, and 2.5%.
In addition, the study found that even with increased IHR, hospitals required over ten days more to detect a new variant compared to sampling a percentage of travelers arriving in England.
The study simulated the earliest time for detecting an imported novel variant by testing a community group. The time for detection decreased by three weeks when the community cohort size was increased from 0.04% to 0.36% of the community population.
The earliest time to detect the sample size of the existing community in England, consisting of approximately 140,000 tests conducted every two weeks, was 157 days.
Conclusion
The study findings showed that sampling only 10% of inbound travelers for surveillance could detect the first case of an imported novel variant of SARS-CoV-2 in England 26 days earlier than existing community surveillance.
A 50% increase in the sampling fraction of travelers could result in a speed benefit of 43 days. Sampling 10% of inbound travelers, according to IHR, could detect a variant faster than surveillance testing of hospital admissions by 11 to 19 days.
International collaboration can be useful in achieving high global coverage of surveillance, which can inform national approaches to surveillance. This can also give governments more time to formulate policy decisions in response to new variants of SARS-CoV-2.

*Important notice: medRxiv publishes preliminary scientific reports that are not peer-reviewed and, therefore, should not be regarded as conclusive, guide clinical practice/health-related behavior, or treated as established information.