Sponsored Content by OXGENEMay 20 2020
Every scientist wants to make an impact, though very few are able to identify a single experiment of theirs which led to the establishment of an entire scientific field.
In early 1973, Frank Graham set out to alter human embryonic kidney (HEK) cells with adenoviral DNA, hoping to learn why some adenoviruses caused cancer while some did not. He was certainly not planning to prompt the creation of a brand new field of medical research with a market value of several billion dollars - but that is precisely what he did.
Frank had moved from Canada three years prior to starting his postdoctoral research, working alongside Professor Alex van der Eb at the University of Leiden in the Netherlands. Before that time, he had not been especially involved in the study of adenoviruses, but, working with a postdoctoral supervisor who was already involved in that area resulted in him shifting his focus.
I decided to investigate the correlation between low GC content in adenoviral DNA and oncogenicity. At that time, nobody knew much about how or why cancers started, so using oncogenic viruses to transform normal cells into cancerous cells in culture seemed a good in vitro model for tumour induction.
Frank Graham, McMaster University
Graham continued, “Perhaps it sounds naïve now, but given the little we understood back then, seeking to understand what made some viruses able to initiate this transformation while others couldn't seemed a reasonable way to go about understanding the root causes of cancer.”
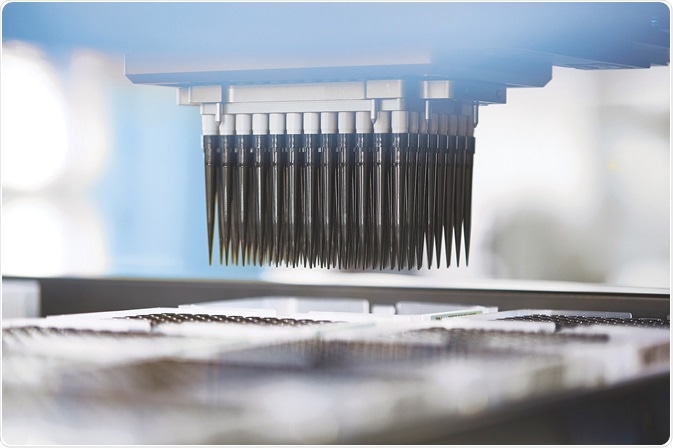
Industry-leading technology and cutting edge automation is central to OXGENE's cell line development workflow.
Graham’s first efforts to transform HEK cells took place in 1972. He initially transfected 40 dishes of cells, but just one produced even a single transformed colony, which, sadly, failed to grow.
1973 saw more success, however, with the identification of a pair of transformed colonies. These were nurtured such that one started to grow, although very slowly. After a six month period, enough cells had been grown to allow a handful of vials to be frozen. “The cells grew slowly – doubling once every 7–10 days – for nearly a year.” But, disaster was not far away.
Back from the Brink: An Immortal Cell Line Emerges
The cells entered a classical crisis, failing to grow for 2 - 3 months but with no apparent reason. Several years later, Graham's spouse discovered a possible reason.
“My wife, Silvia Bacchetti, discovered that pre-crisis HEK293 cells didn't express telomerase,” Graham said. The telomerase enzyme stops telomeres (the repetitive DNA at chromosome tips) from shortening with each cell division. “Without telomerase, the telomeres get shorter and shorter with every division until eventually, they reach a critical length and the cell dies.”
Hardly any of the cells survived the crisis. “Silvia's research demonstrated that these post-crisis cells did express telomerase, which suggested that the transition to and through crisis selected for cells producing this enzyme, and potentially other growth-promoting genes too,” Graham explains. Regardless of what caused this phenomenon, after the crisis had passed, the cells' growth rate increasing, doubling every 2 - 3 days. At this point, Graham had, at least, created an immortal, adenovirus-transformed human cell line.
Laying the Foundations of Gene Therapy
Graham later returned to Canada to join McMaster University in Ontario. Here he resumed his research into adenoviruses, concentrating first on exploring the molecular biology of cell transformation before shifting to working to understand the development of adenoviruses as vectors for mammalian gene transfer.
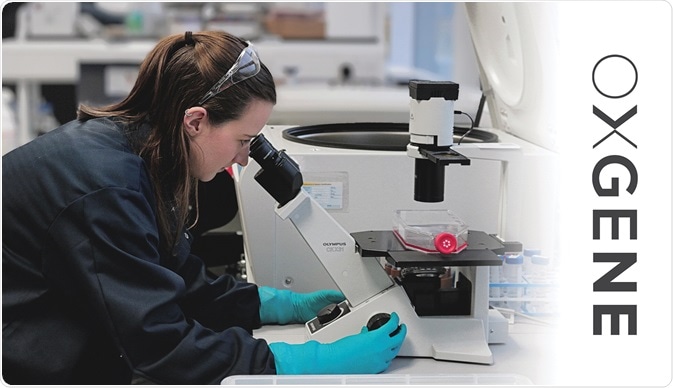
OXGENE's scientists adapted a parental pool of HEK293 cells to grow in different media, before screening thousands of single-cell clones for optimum AAV production.
Graham's HEK293 cells offer a key advantage for this particular research - the transformation of these cells via adenoviral DNA means that they may support the growth of adenoviruses which have been modified so that they no longer self-replicate. This is to say an altered virus is unable to grow outside or HEK293 cells, or other types of cell which support adenoviral replication.
Support from the missing viral DNA (now part of the cells' genome) allows the virus to continue to grow. Additionally, the adenovirus can be further modified to incorporate human gene products such as standard copies of disease genes.
Once enough of the virus has been grown in HEK293 cells, these may be transferred into patients who have genetic diseases. Once they have reached the patients, the virus will express the correct - or therapeutic if appropriate - copies of the gene, thus counteracting any effects of the initial mutation or treating a disease. However, these cells are unable to replicate, making them unable to cause disease themselves.
This is, essentially, the central premise of gene therapy, accounting for most of the commercial interest in HEK293 cells.
A Cure and a Flaw
Up until the late 1980s and early 1990s, the majority of research with Graham's cells was academic, as he had generously shared them within the academic community. However, it did not take long for companies to begin contacting him about potential commercial uses for HEK293 cells.
While gene therapy was still a relatively new area, by the mid-1990s it's commercial potential had become clear. Researchers believed that it would ultimately lead to cures for almost every genetic disease.
During this period, most gene therapy research involved the use of adenoviral vectors. Georg Roth, the principal at Roth Bioprocess Consulting and a former Senior Scientist at Berlex - which was an early licensee of HEK293 cells – said that adenovirus vectors were, “the workhorse of gene therapies.” These vectors offered straightforward handling as well as a good safety profile, but it was only possible to manufacture them using HEK293 cells.
“Unfortunately,” Roth continued, “these cells had a serious flaw.” HEK293 cells express the adenoviral gene E1, which permits adenoviruses lacking this gene to grow. However, when Graham first transformed these cells, molecular biology techniques were not especially advanced.
“The fragment of viral DNA in 293 cells comprises sequences from the extreme end of the genome and extends across E1 and beyond.” The cells, therefore, contained adenoviral DNA sequences flanking the viral genes that are typically deleted in adenoviral vectors. “This overlap allows homologous recombination to occur.”
In some cases, the replication-incompetent adenoviral vector was able to force out the therapeutic gene being carried, reincorporating the E1 gene from the HEK293 cells, leading to a wildtype virus.
This presented a considerable safety issue for gene therapies - while a patient will receive the therapeutic gene they need, there is also the risk of adenoviral infection. Thankfully, different viral vectors – especially adeno-associated virus (AAV) and lentiviral vectors – are able to mitigate this risk. This is because these vectors do not have this problem with homologous recombination.
The Fall of the Adenoviral Vector for Single-Gene Disorders
After a number of years of research, it became increasingly clear that adenoviruses were not the whole solution for successful gene therapy applications. These viruses were prone to provoking an immune reaction while failing to integrate the therapeutic gene into the target cell. This resulted in them only delivering a transient response, rather than a long-term cure for genetic disease.
1999 saw the advent of a major critical setback for adenoviral-based gene therapies. Teenager Jesse Gelsinger sadly died during a clinical trial at the University of Pennsylvania which had sought to correct an X-linked genetic disease of the liver.
Following this incident, adenovirus-based gene therapy trials were suspended immediately, and it appeared for a number of years the industry would never recover.
Scientists realized that openness and transparency with the public was the best approach, is especially clear about the challenges of gene therapies. This improved dialog and public awareness allowed progress to resume in an exceptionally promising area of medical research.
At this point we set up the British Society of Gene Therapy. We knew that it was important to engage with the public about what had happened and focus on the potential benefits that might still be achieved.
Len Seymour, Professor of Gene Therapies, University of Oxford
Establishing the Potential for Gene Therapies
Around twenty years ago, Seymour highlighted three major goals with gene therapies. The first was oncolytic (cancer-killing) viruses. “That – alongside using adenoviruses as a vector for vaccines – is where adenoviruses are coming back into their own again now.” Graham agrees with this sentiment, adding, “Personally, I think vaccine development is the most important application for adenoviral vectors.” The second application is gene supplementation therapies – the traditional gene therapy or replacement concept. The last goal was that of cell therapies, like the new CAR-T therapies. “In cell therapies, you take cells from the patient, modify them in some way, and then inject them back in.”
The renewed potential of gene therapy had become obvious by 2010, with HEK293 still being central to the manufacturing of the viral vectors typically employed to deliver replacement genes. At Oxford, scientists working in the University's Clinical Biomanufacturing Facility were able to adapt suspension HEK293 cells to serum-free suspension culture.
Graham's initial HEK293 cells were grown in adherent culture, meaning they were attached to the bottom of a cell culture dish. This is acceptable in many cases and is actually how cells generally prefer to grow. Grown in this way, cells can remain in close contact with their neighbors, mimicking the formation of tissues within an organism.
For gene therapies that require enormous numbers of cells to produce the quantity of virus needed for clinical manufacture, however, the physical space involved in culturing this number of adherent cells is a major limitation.
Adapting cells so that they are suitable for growth in suspension is a key factor in the scaling of gene therapies for clinical and commercial manufacture. Further modifying the cells to enable growth without animal serum is also important to take cells into clinical production.
Scalable Technologies for Gene Therapy Manufacture
The gene therapy market had recovered by 2016 and was seeing significant progress in each of the three areas that Seymour had predicted decades ago.
Adenoviruses are now commonly utilized as oncolytic viruses, or as vaccines for a number of diseases. These are largely passed by for gene supplementation therapies, though. AAV and lentiviral vectors are used much more widely in these areas, with both produced using HEK293 cells.
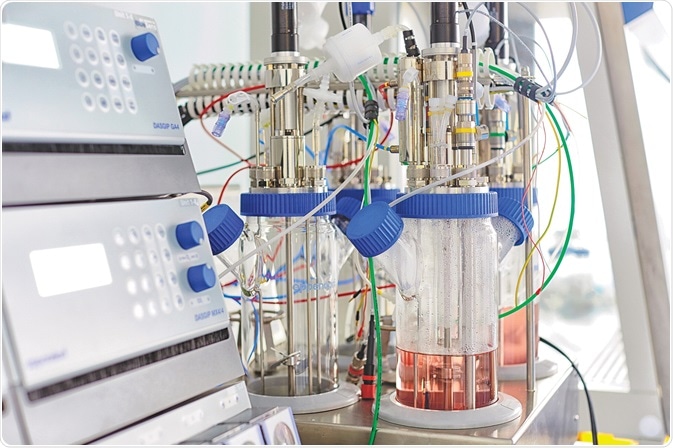
OXGENE's ambition is to pioneer the development of carefully optimized technologies for scalable, cost-effective, and high-quality gene therapy manufacture.
Oxford Genetics – now known as OXGENE™ – licensed the cells from Oxford University in order to take a cell line which had been a cornerstone of the global gene therapy market since its inception and further optimise it to maximize viral yield.
One of the major challenges of gene therapy nowadays is scaling manufacture. At the moment, it's difficult – and very expensive – to produce the amount of virus necessary to treat a patient, especially one with a systemic disease. Those costs are unfortunately passed down to the patient, or the health providers. Right now, a course of gene therapy treatment can cost over $2 million. That's clearly unsustainable. We want to find a way to make gene therapies more cost effective, and scalable manufacture is a big part of that.
Qian Liu, Senior Group Leader
HEK293 cells are a fundamental part of OXGENE's technologies. “One of our aims is to enable efficient manufacture of AAV, for example, serotype AAV5.” OXGENE scientists have modified the parental cell line for growth in a wide range of media types. All media must supply the energy, nutrients, and growth factors required for the cells to survive and function.
Different forms of media have the potential to change the cells' growth rate and/or their gene expression profile. For example, one specific type of media was found to be optimal for AAV5 production.
“Next we sorted the media-adapted parental cell population into single cells and selected the clones that grew fast and produced the most rAAV5,” Liu outlines. “This same clone was also good at producing lentivirus too, and a few other AAV serotypes. Once we'd properly established our clonal cell line, we started the process of GMP banking it.”
GMP, which stands for Good Manufacturing Process, is a certification process whereby cells are manufactured in a controlled, highly regulated fashion before being extensively tested for any pathogens and contaminants. This certification is imperative if the cells are to be employed in any clinical trial or therapeutic application.
“We're extremely excited to have GMP-banked our optimized HEK293 clonal cell line,” says Liu. “I love the idea that we can trace these cells all the way back to Frank's experiment in 1973. It's funny to think that without these cells, the gene therapy field might never have opened up the way that it has. Now we hope that our cells will be central to the next part of the process too – making gene therapies scalable, cost-effective, and accessible to everyone who needs them.”
About OXGENE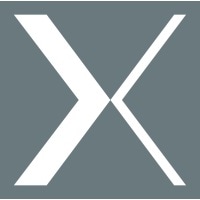
OXGENE™ combines precision engineering and breakthrough science with advanced robotics and bioinformatics to accelerate the rational design, discovery and manufacture of cell and gene therapies across three core areas: gene therapy, gene editing and antibody therapeutics.
Gene therapy: We’re transforming the vision of truly scalable gene therapies into a reality; progressing our industry leading transient gene therapy systems towards alternative technologies for scalable, stable manufacturing solutions.
Gene editing: We have automated gene editing to deliver CRISPR engineered cell lines at unparalleled speed, scale and quality and generate complex disease models in mammalian cells.
Antibody therapeutics: We’re employing a novel proprietary mammalian display technology to discover antibodies against previously intractable membrane proteins.
OXGENE™ works at the edge of impossible in mammalian cell engineering. Our scientific expertise and technology solutions address industry bottlenecks. For more information, please visit www.oxgene.com
Sponsored Content Policy: News-Medical.net publishes articles and related content that may be derived from sources where we have existing commercial relationships, provided such content adds value to the core editorial ethos of News-Medical.Net which is to educate and inform site visitors interested in medical research, science, medical devices and treatments.