Sponsored Content by Bruker BioAFMReviewed by Mychealla RiceDec 22 2022
Atomic force microscopy (AFM) has become an indispensable technique for examining various samples with nanoscale resolution under physiological conditions. In addition to topographic measurements, information regarding the interaction and mechanical characteristics, such as adhesion and elasticity, has recently been extracted using AFM.1
Novel developments also focus on quick and high-speed AFM to improve temporal resolution.2 The combination of AFM with optical microscopy has expanded the number of possible applications, particularly when specificity is needed.3, 4
Super-resolution light microscopy, such as AFM, has received significant interest from the scientific community in the last decade, leading to the invention of various far-field microscopy methods that allow researchers to resolve objects below Abbe’s diffraction limit. In 1994, Hell and Wichmann presented the principle of stimulated emission depletion (STED) to overcome the diffraction limit.5
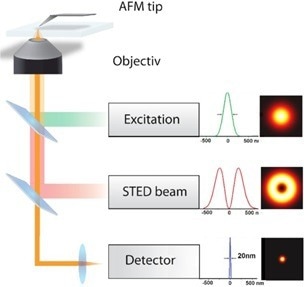
Figure 1. Scheme of an AFM and STED based system showing the major components. The sketch includes the excitation source, the STED beam, the detection unit and the AFM. Image Credit: Bruker BioAFM
The central concept of STED microscopy is to limit the effective fluorescence region by selectively turning off fluorophores at the rim of the focal spot. Therefore, the sample is scanned with an excitation focus and a donut-shaped STED focus. The STED light turns off all fluorophores except those inside a nanometer-sized central area.
Spontaneous fluorescence can only occur inside this zone. STED microscopy has been steadily enhanced since its experimental viability was first shown in 1999, and is now a standard method in optical microscopy.6-9
The 2014 Nobel Prize in Chemistry highlighted the significance of Stefan Hell’s invention and the technical execution of STED microscopy.
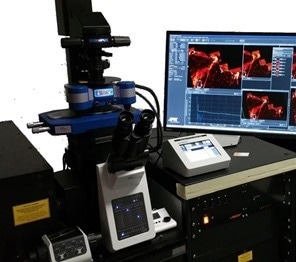
Figure 2. JPK NanoWizard AFM integrated into an Abberior Instruments Expert Line STED microscope (as an example the NanoWizard ULTRA Speed is shown). Image Credit: Bruker BioAFM
In recent years, there has been a surging trend toward integrating microscopy methods, such as AFM and super-resolution. This trend is fueled by the capability to integrate readouts from both microscopy techniques in a single experiment and generate datasets with a much greater information density. Moreover, improved accessibility and simplified usage concepts of AFM and STED microscopes have made their integration easier.
In AFM, new modes such as QI mode and simple features enable novices to generate high-quality data on topography, adhesion, and mechanical characteristics. New lasers and concepts such as easySTED have allowed the construction of highly durable super-resolution microscopes in STED microscopy, paving the way for their usage in routine applications.
Integrating different microscopy methods opens new avenues for examining biological samples in physiological settings, resulting in versatile information output.
Correlative nanoscopy: AFM-STED
In biological samples, atomic force and STED microscopy have a comparable nanoscale resolution. However, both strategies have their restrictions and disadvantages. Combining both methods is preferable for developing a more comprehensive dataset and acquiring a better understanding of the specimen under investigation.
AFM is a surface-examining method. AFM can also be utilized as a force sensor for detecting interaction forces or mechanical characteristics due to its nanometer-scale resolution. Moreover, AFM is also useable as a nanomanipulator with Piezo-controlled, sub-nanometer movement capabilities.10
AFM is still a surface technique and is always confined to analyzing the sample’s top layer. AFM also lacks chemical specificity. There are approaches such as AFM recognition (using functionalized cantilevers for antigen-antibody interaction) and tip-enhanced Raman spectroscopy that offer optimal chemical specificity, but these techniques are not practical because of their time-consuming analysis.
Combining AFM with optical/fluorescence microscopy is the most effective method for gaining access to chemical recognition and expanding AFM capabilities.11
Since the beginning, optical integration has been a primary objective for JPK Instruments AG. The JPK NanoWizard AFM is well-suited for use with optical microscopes due to its tip-scanning design. The sample stays stationary while the AFM is operating.
The AFM head is designed to eliminate optical path disruption. Crosstalk between the AFM laser and the optical signal is avoided by filters inside the AFM head, allowing the two strategies to work together.
Although combining AFM and traditional (diffraction-limited) light microscopes is common, it suffers from a resolution mismatch between the two techniques. Diffraction limits the resolution of ordinary light microscopes to roughly 220 nm in the image plane (XY).
This mismatch can be addressed by STED microscopy's greatly enhanced resolution of around 10 nm. Abberior Instruments’ microscope product lines are compatible with current microscope bodies and experimental settings due to their optimal design. This provides the ideal environment for correlative nanoscopy.
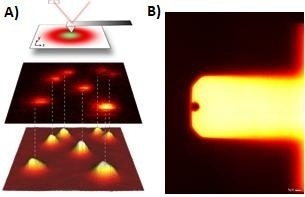
Figure 3. A) STED + AFM principle. STED and AFM images of fluorescence beads as well as correlation of both images are shown.12 B) Reflection image of Nanoworld USC cantilever. Image Credit: Bruker BioAFM
Since 2011, JPK Instruments AG has worked consistently on combining AFM and super-resolution techniques.12 To achieve perfect optical integration, JPK has significantly expanded the adaptability of its revolutionary DirectOverlay feature.
Calibration is performed automatically using known cantilever positions and offsets to translate the optical image into the AFM coordinates. The calibrated optical image is transferred into the JPK SPM software, where scan regions within the optical image can be selected.
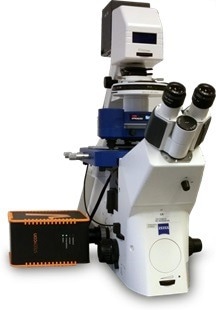
Figure 4. JPK NanoWizard AFM integrated into Abberior Compact line – STEDYCON (as an example, the NanoWizard 4 BioScience is shown). Image Credit: Bruker BioAFM
A sample of two separate fluorescent bead species with different colors was evaluated using a JPK NanoWizard and an Abberior Expert Line STED microscope with two excitation lasers (561/ 640 nm) and a STED laser with a wavelength of 775 nm in a first proof-of-principle experiment.
The beads have a diameter of 40 nm and may be recognized using two-color STED microscopy. Crimson beads (640 nm excitation laser) are displayed in red, while red fluorescent beads (RFB) (561 nm excitation laser) are shown in green.
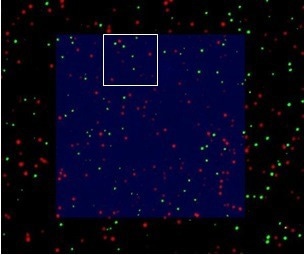
Figure 5. Overlay of AFM and STED image. Scan size: 5 µm x 5 µm. Image Credit: Bruker BioAFM
DirectOverlay was evaluated for testing the integration of STED and AFM images, and the overlay precision within a 20 x 20 µm scan range of greater than 15 nm was recorded. The STED signal in the scan region correlates well with the AFM image (figure 5).
A narrower scan region was used to ensure that all of the beads detected in AFM could be seen in the STED image (figure 6). As indicated, the overlay of both measurements matches quite well. However, at certain points, a bead observed in the AFM analysis is not present in the STED measurement (figure 6A).
A 3D topographical examination of the bead diameter reveals that the beads vary in size, with some being as small as 25 nm. Moreover, some beads do not have a fluorescent label and can only be detected in the AFM images.
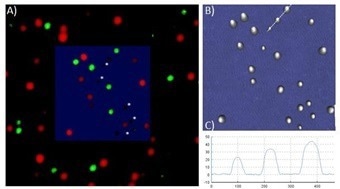
Figure 6. A) Overlay of AFM and STED image. Scan size: 1 µm x 1 µm. Beads measured in AFM that do not correspond to a STED signal are marked with a white star. B) AFM topography image in 3D clearly shows the divergent distribution of bead diameter C) Cross section of three beads. Image Credit: Bruker BioAFM
Although the experiment is relatively straightforward, combining both techniques offers additional information about the sample quality. A correlative method supplements and enhances data obtained through parallel procedures.
Simultaneous AFM and STED of living fibroblasts – Nanomanipulation
The NanoWizard AFM was utilized in conjunction with an Abberior Instruments Expert Line STED microscope in this experiment. Living human skin fibroblasts were labeled with SiR Tubulin (Silicone incorporating Rhodamine with docetaxel as a tubulin-binding moiety) and imaged in cell culture media for these measurements.
The AFM tip modified the labeled microtubules while the same region was scanned in STED mode to demonstrate the feasibility of real simultaneous measurement.
The STED microscope vividly monitors the bending, manipulating, and rupturing of microtubules by the AFM tip in real time. Due to the DirectOverlay feature, microtubules can be targeted and modified on the nanometer scale.
The forces applied by the AFM tip can also be monitored and regulated. Figure 7 depicts a time series of the modification and a rupture of certain microtubules inside the cell. Adapting the modification force to stretch specific microtubules is also possible, providing further information about the elasticity of microtubules.
In addition to scratching or moving the sample, imprinting a cell or administering a local stimulation is possible. For a stimulus investigation, actin filaments were labeled with SiR Actin (Silicone containing Rhodamine with jasplakinolide as an actin-binding moiety).
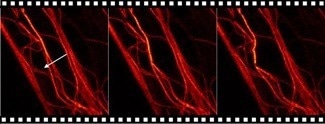
Figure 7. Time series of AFM manipulation-STED measurement. Microtubules labeled with SiR Tubulin and imaged with an Abberior Instruments Expert Line STED microscope (excitation, 640 nm; STED, 775 nm). The AFM manipulation path is marked with an arrow. The manipulation force was set to 5 nN with a velocity of 1 µm/s. Image Credit: Bruker BioAFM
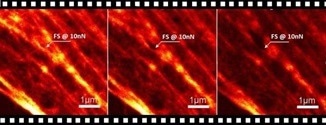
Figure 8. Time series of AFM stimulation-STED measurements. The actin cytoskeleton was labeled with SiR Actin and imaged with an Abberior Instruments Expert Line STED microscope (excitation, 640 nm; STED, 775 nm). The AFM stimulation position is marked with an arrow. Image Credit: Bruker BioAFM
Abberior Instruments’ patented RESCue STED imaging option was employed to prevent bleaching in live cell STED studies. In this experiment, a 10 nN force was selectively applied to the fibroblast cell.
The stimulus parameter was designed using the JPK Force RampDesigner. Three seconds of continuous stimulation were followed by one second of relaxation (no force applied). The alteration of the actin filament structure was observed via STED.
Local mechanical stimulation is known to cause a reorganization of the cytoskeleton and focal adherence.14,15 However, following actin restructuring, polymerization, and depolymerization, in connection with a nanoscale-precise mechanical stimulus with high optical sensitivity, has been challenging.
Figure 8 depicts the impacts of AFM stimulation on the development of actin fibers. Applying a 10 nN force alters various actin filament structures, allowing the user to see the kinetics and restoration of the actin filament architecture.
Living fibroblasts – Nanomechanical investigation
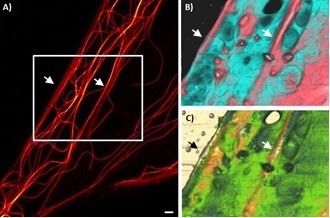
Figure 9. Living human skin fibroblast. A) Microtubules labelled with SiR Tubulin and imaged with Abberior Instruments Expert Line STED B) 3D topography AFM image, 12.5 µm x 9 µm; range: 1 µm C) 3D topography image overlaid with Young’s modulus range: 60 kPa. Image Credit: Bruker BioAFM
Using JPKs QI mode, the mechanical information of live fibroblast cells was also assessed. AFM can produce a high-resolution image of surface topography and may determine mechanical properties such as elasticity and adhesion.
In STED microscopy, recording a 3D stack to comprehend the underlying intracellular functions is conceivable. The integration and superimposition of AFM and STED images enable the correlation of disparate data and the comprehension of how the morphological features originate from the underlying cytoskeleton fibers. Figure 9A shows the microtubule distribution with high resolution in the STED image.
A distinctive portion of the cell was chosen for AMF to correlate the surface topography and the elasticity imaging with the microtubule distribution.
The STED image depicts two sections, parallel and close-packed microtubule bundles (marked with an arrow). The AFM images can also identify these regions. The two parallel regions appear higher in the topographical image and stiffer in Young’s modulus image. Additionally, distinct vesicle/particle-like entities can be recognized on the cell surface.
Although certain structures appear softer than the surrounding cell membrane, other particle-like structures are stiffer. Living cells are in continual contact with their environment.
The cell membrane is dynamic, undergoing re-modulation, endocytosis, and exocytosis. To learn more about these processes, it is possible to use AFM high-speed measurements with the NanoWizard ULTRA Speed or ULTRA Speed A to track cell dynamics and cytoskeleton remodeling.
Nanomechanical information was also linked with actin-labeled cells. The findings are shown in figure 10. Thicker actin filament bundles may be detected as a stiffer region in Young’s modulus image. Different actin filaments can also be observed in the height image.
The combined technique of STED and AFM allows the correlation of information on chemical recognition, intracellular features, and topography/nanomechanical characteristics with nanoscale precision. As a result, it is a strong instrument for studying cells and biological specimens.
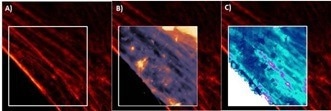
Figure 10. Living human skin fibroblast. A) Actin labeled with SiR Actin and imaged with Abberior Instruments Expert Line STED B) Topography AFM image, 15 µm x 15 µm; range: 0.7 µm C) 3D topography image overlaid with Young’s modulus range: 90 kPa. Image Credit: Bruker BioAFM
Conclusion
Correlative nanoscopy is a powerful tool for biological applications, as shown by the performance, dependability, and advantages of a combined AFM and STED. Correlative microscopy delivers supplementary information and improves data because of its capacity to make measurements concurrently.
Super-resolution light microscopy offers molecular selectivity and intracellular information to the AFM data, while AFM provides 3D surface imaging and nanomechanical information.
Experiments using nanomanipulation revealed the ability to conduct simultaneous measurements on all levels. The measurements shown on living human skin fibroblasts are the first examples of essentially limitless applications for novel and innovative correlative techniques.
Sample courtesy
The proof-of-concept experiment with two distinct types of beads, the nanomanipulation experiment and the analysis of living human skin fibroblast, was carried out in partnership with Abberior Instruments GmbH. Abberior Instruments GmbH provided the samples for these experiments.
References and Further Reading
- Formosa-Dague, C.; Speziale, P.; Foster, T. J.; Geoghegan, J. A.;Dufrene, Y. F. Zinc-dependent mechanical properties of Staphylococcus aureus biofilmforming surface protein SasG. Proc.Natl.Acad.Sci. 2016,113, 410−415.
- Casuso, I.;Rico, F.; Scheuring, S. High-speed atomic force microscopy: Structure and dynamics of single proteins, Curr. Opin. chem biol, 2011: 15(5), 704-709.
- Chiantia, S.; Kahya, N.; Ries, J.; Schwille, P. Effects of Ceramide on Liquid-Ordered Domains Investigated by Simultaneous AFM and FCS, Biophysical Journal, 2006: 90(12), 4500-4508
- Dufrêne, Y.F.; Ando, T.; Garcia, R.; Alsteen, D.; MartinezMartin, D.; Engel, A.; Gerber, C.; Müller, D.J. Imaging modes of atomic force microscopy for application in molecular and cell biology, Nat Nanotechnol. 2017: 12(4), 295-307
- Hell, S.W.; Wichmann, J. Breaking the diffraction resolution limit by stimulated emission: stimulatedemission-depletion fluorescence microscopy. Opt Lett. 1994: 19, 780–782.
- Klar, T. A.; Hell S.W. Subdiffraction resolution in far-field fluorescence microscopy, Optics Letters, 1999: 24(14), 954–956.
- Klar, T.A,; Jakobs, S.; Dyba, M.; Egner A.; Hell S.W. Fluorescence microscopy with diffraction resolution barrier broken by stimulated emission. Proc Natl Acad Sci 2000: 97, 8206–8210.
- Hell S.W. Far-field optical nanoscopy. Science. 2007: 316, 1153–1158
- Harke, B.; Keller, J.; Ullal, C.K.; Westphal, V.; Schönle, A.; Hell, S.W. Resolution scaling in STED microscopy. Opt Express. 2008: 16(6), 4154-4162
- Chacko, J.V.; Canale, C.; Harke, B.; Diaspro, A. SubDiffraction Nano Manipulation Using STED AFM. PLoS ONE 2013: 8(6), e66608
- Super-Resolution imaging in Biomedicine edited by Diaspro, A.; van Zandvoort M.A.M.J., CRC Press 2017
- Harke, B.; Chacko, J.V.; Haschke, H.; Canale, C.; Diaspro, A. A novel nanoscopic tool by combining AFM with STED microscopy Opt Nano 2012: 1 (3)
- Monserrate, A.; Casado, S.; Flors, C. Correlative Atomic Force Microscopy and Localization-Based SuperResolution Microscopy: Revealing Labelling and Image Reconstruction Artefacts. ChemPhysChem, 2015: 15, 647–650.
- Lim, S. M.; Trzeciakowski, J. P.; Sreenivasappa, H.; Dangott, L. J.; Trache, A. RhoA-induced cytoskeletal tension controls adaptive cellular remodeling to mechanical signaling. Integrative biology, 2012: 4(6), 615- 627.
- Charras, G.T; Horton, M.A, Single Cell Mechanotransduction and Its Modulation Analyzed by Atomic Force Microscope Indentation. Biophysical Journal 2002: 82(6) 2970–2981
About Bruker BioAFM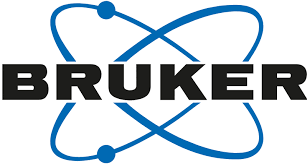
Bruker BioAFM, former JPK Instruments AG, is a leading manufacturer of nano-analytical instruments - particularly based on atomic force microscope (AFM) and optical tweezers systems - for life sciences and soft matter applications.
We combine the highest technical skills with visionary applications. Our work applies nanotechnology in ways to provide solutions to challenges facing researchers in life sciences and soft matter today. Driven by inspiration and ambition, it is our conviction that only the best tools are good enough for the research of life. We are listening with the ear of a scientist in detail to the current challenges of our customers and find individual solutions for individual problems. This is how we understand our business.
Sponsored Content Policy: News-Medical.net publishes articles and related content that may be derived from sources where we have existing commercial relationships, provided such content adds value to the core editorial ethos of News-Medical.Net which is to educate and inform site visitors interested in medical research, science, medical devices and treatments.