Ribonucleic acid (RNA) is a form of nucleic acid, one of the four primary macromolecules required for all known forms of life.
As a polymeric molecule, RNA is made up of chains of nucleotides, with each nucleotide composed of a ribose sugar group (DNA features deoxyribose), an attached phosphate group, and one of four nitrogenous bases.
Three of these four nitrogenous bases—adenine, cytosine, and guanine—are also found in DNA. While DNA contains thymine, RNA includes uracil.
RNA is key to the majority of biological processes, with its functions categorizable into two primary roles:
- RNA can function as a messenger, encoding instructions from DNA and passing these to the ribosome for protein synthesis (messenger RNA).
- RNA performs a given function itself (non-coding RNA, or ncRNAs).
Human genome and transcriptome sequencing show that approximately 76 % of the genome is transcribed, but only 2 % of this codes for proteins.
The function of a large proportion of the human genome remains unknown, but it is now clear that this 98 % is not “junk DNA,” as was initially theorized.
These ncRNAs perform a wide range of functions and are frequently involved in housekeeping activities. They also play vital regulatory roles in many areas of development and disease.

Image Credit: Norgen Biotek Corp.
RNA’s role in protein synthesis
RNA’s primary role was initially believed to be protein creation. Proteins are the cell’s primary workhorses, involved in transporting molecules, catalyzing reactions, and providing structure.
Three main types of RNA are involved in protein synthesis: mRNA, tRNA, and rRNA.
mRNA is synthesized by the enzyme RNA polymerase. This occurs by using the sequence of bases in a DNA strand to create a complementary mRNA strand. This pre-mRNA is then processed—spliced, possibly edited, capped, and polyadenylated—to increase its stability and expand the diversity of proteins it can code for. The mRNA then moves to the ribosome.
The ribosome features two subunits, with each subunit composed of an rRNA molecule and its associated proteins. Another RNA species becomes involved once the mRNA enters the ribosome: tRNA, which helps deliver amino acids to the ribosome.
Nucleotides in the mRNA work together to dictate the order of the amino acids. The tRNA carries the amino acids, while the rRNA molecules forming the ribosome assemble them into the final protein.

Image Credit: Norgen Biotek Corp.
Effective methods for cholera prevention

Image Credit: Norgen Biotek Corp.
Small non-coding RNA: snRNA and snoRNA
Research into other small ncRNAs (sncRNAs) has expanded this simplified view of the central process in recent years.
While it was historically understood that DNA gives rise to protein via the functions of mRNA, rRNA, and tRNA, closer inspection has shown that protein synthesis and other housekeeping functions require a wider range of RNA species, including small nuclear RNA (snRNA) and small nucleolar RNA (snoRNA).
Small nucleolar RNAs (snoRNAs)
Significant numbers of small nucleolar RNAs (snoRNAs) are found in the nucleoli of eukaryotic cells. These small non-coding RNAs have a length of 60–300 nt and are encoded by the intronic regions of both protein-coding and non-protein-coding genes.
The primary function of snoRNAs lies in the complex chemical modifications undergone by pre-RNAs before they become mature rRNAs. snoRNAs feature specific sequences that guide them to particular cellular RNAs (rRNA or snRNA). This allows them to form a complex with around four other proteins, resulting in RNA-protein complexes called snoRNPs.
The snoRNP is understood to catalyze either 2′-O-methylation or pseudouridylation modifications, but new research is also exploring its roles in the regulation of alternative splicing, the regulation of mRNA levels, and the modification of N4-acetylcytidine (ac4C).3
These regulatory functions also impact cell development. Organismal complexity increases in line with the number of snoRNA genes. For example, one study estimated that there are 76 snoRNAs encoded in 64 transcription units in the yeast Saccharomyces cerevisiae, while the number of snoRNAs is estimated at around 550 in humans.4
Small Cajal body-specific RNAs (scaRNAs) are a new subgroup of snoRNAs that localize only in the Cajal body.
snoRNAs have been linked to a range of cancers, with recent research revealing that SNORD1C is highly expressed in colorectal cancer (CRC). Knocking down SNORD1C inhibited tumor growth in vivo.
Additionally, the expression of SNORD1C in the serum of CRC patients was considerably higher than in controls, making it a potential biomarker when found in liquid biopsy samples.
Research has also revealed that a variety of different snoRNAs are linked to lung cancer, leukemia, ovarian cancer, breast cancer, and hepatocellular carcinoma.5
Small nuclear RNA (snRNA)
snRNAs are also involved in the housekeeping function of translation, most notably in splicing. These nucleosomal RNAs are localized in the eukaryotic nuclei alongside the Cajal bodies and splicing speckles.
snRNA works with proteins to form small nuclear ribonucleoproteins, or RNA-protein complexes called snRNPs (pronounced "snurps").
The most commonly encountered human snRNA components are U1 spliceosomal RNA, U2 spliceosomal RNA, U4 spliceosomal RNA, U5 spliceosomal RNA, and U6 spliceosomal RNA. The nomenclature of these components stems from their high uridine content.
The snRNAs in the snRNP complex make the splicing process more specific by recognizing the sequences of critical splicing signals at the 5' and 3' ends, as well as at the branch site of introns.
Like those found in rRNA, the snRNAs in snRNPs perform both structural and enzymatic roles. snRNAs feature a variety of modifications, many of which are performed by snoRNAs.6
The process of pre-mRNA splicing removes introns and stitches together exons to create mature mRNA. Ten percent of all disease-causing single-point mutations in humans result in splicing defects, highlighting the importance of fidelity in this process.
snRNPs have also been implicated in diseases such as medulloblastoma, spinal muscular atrophy, dyskeratosis congenita, and Prader–Willi syndrome, the latter of which has been linked to the deletion of a region of paternal chromosome 15 that includes a brain-specific snRNA able to target the serotonin-2C receptor mRNA.7
Scientists are harnessing the sequence-specific splicing function of snRNAs as therapeutic agents. For instance, Locana Bio is using AAV gene therapy vectors to deliver genetically engineered snRNAs with binding sites relevant to disease alleles. These can be used to restore protein production in frameshift mutations or to splice out detrimental exons.8
Regulatory short non-coding RNAs
snRNAs and snoRNAs are involved in the translation process via the processing, modifying, and splicing of RNA, but they also have other regulatory roles. For example, some ncRNAs are more directly involved in the differential regulation of gene expression.

Image Credit: Norgen Biotek Corp.
MicroRNA (miRNA)
In 1993, microRNAs were recognized as small non-coding RNAs with a regulatory function in a forward genetic screen of C. elegans. miRNAs have an average length of 22 nucleotides and are exported from the nucleus as a hairpin structure that folds back on itself.
The Dicer enzyme trims this pri-miRNA, and once the other strand is discarded, the miRNA recruits the Argonaute protein to form an RNA-induced silencing complex (RISC).
The single-stranded miRNA is responsible for its specificity, silencing the target mRNA through base pair complementarity. This miRNA-mRNA pairing is generally entirely complementary in plants but only partially complementary in animal cells.
In most instances, miRNA suppresses mRNA expression by binding to the 3′ untranslated region (UTR) of its target mRNA in a sequence-specific manner. This results in translational inhibition and some degree of mRNA instability.
Full complementarity between the miRNA and its target mRNA is not required, meaning that a specific miRNA can target multiple mRNAs, generally regulating multiple genes within a common pathway.
It is currently believed that over a third of the mRNAs in the mammalian genome are regulated by at least one miRNA, with some mRNAs regulated by multiple miRNAs.
miRNAs are central to many normal developmental pathways, having been implicated in the development of most organs and cells. It is also important to note that miRNAs are interwoven into transcriptional and signaling networks, and anomalous expression has been linked to a wide range of diseases.
For example, chronic lymphocytic leukemia was the first human disease found to be caused by deregulation of miRNA,9 with many other miRNAs later linked to cancers. This led to the coining of the term “oncomirs.”
Expression levels of some miRNAs have been shown to be upregulated in certain cancers, and they can now be used as diagnostic or prognostic biomarkers.
For example, plasma miR-21, miR-494, and miR-1973 represent promising disease response biomarkers in classical Hodgkin lymphoma.10
Not only is research on miRNAs revealing biomarkers of disease, but it is also suggesting new treatment strategies. For example, repairing defective miRNA pathways in tumors to inhibit tumor cell proliferation has become a new strategy for cancer treatment.
Small Interfering RNA (siRNA)
Small interfering RNAs—also called short interfering or silencing RNAs (siRNAs)—are double-stranded RNA molecules approximately 20–24 (typically 21) base pairs in length.
These function similarly to miRNAs, silencing or suppressing mRNA expression, but they are also produced by Dicer processing other RNA. Once Dicer produces the siRNA duplex, additional proteins are recruited to form the RNA-induced silencing complex (RISC).
The Argonaute proteins (Ago) are directly involved in mRNA degradation and represent a key component of RISC.
One functional difference is that siRNAs are highly specific and target a single mRNA, whereas miRNAs can regulate multiple mRNAs. siRNAs prevent translation by degrading mRNA to silence gene expression.
This gene-specific mechanism of knocking down genes by preventing their translation has led to a wide range of applied and basic research.
RNA interference (RNAi) is now regarded as a powerful functional genomics tool, with the potential to be used as a gene therapy approach.
Research into specific delivery mechanisms and minimizing off-target effects is ongoing, but four RNAi-based therapeutics have already been approved by the FDA. Each of these therapeutics uses synthetically delivered siRNA to degrade mRNA linked to a rare genetic disease.11
RNAi differs from CRISPR in that RNAi blocks gene expression at the mRNA level, whereas CRISPR blocks gene expression at the DNA level.
Piwi-interacting RNA (piRNA)
Another class of small non-coding RNA is Piwi-interacting RNA (piRNA). piRNAs represent the most abundant class of small non-coding RNA molecules expressed in animal cells, and these are slightly longer than miRNAs and siRNAs, measuring around 26–31 nucleotides.
piRNA is similar to miRNA and siRNA in that it forms an RNA-induced silencing complex (RISC) and interacts with Argonaute proteins. However, rather than silencing mRNA, piRNA is primarily involved in silencing transposable elements. Some research suggests that piRNA can also regulate DNA methylation
piRNAs are formed from transposon traps and do not rely on Dicer for biogenesis. These traps provide a type of RNA-mediated adaptive immunity against transposon invasions and expansions.
It is also important to note that piRNAs do not possess any secondary structure, and their function is not dependent on sequence specificity.
In mammals, piRNA activity in transposon silencing appears to be most important during embryo development. piRNAs are also necessary for spermatogenesis in both C. elegans and humans.12
tRNA-derived small RNAs (tsRNA)
tRNA-derived small RNAs (tsRNAs) are the most recent additions to the world of small RNAs. These ncRNAs are produced by cleaving mature tRNAs. They function similarly to miRNAs, forming a RISC and having the ability to silence transcription in a sequence-dependent manner.
tsRNAs also indirectly affect gene expression by binding to proteins and acting as sponges. Additionally, they can regulate translation because tsRNA-containing protein complexes can displace the scaffolding protein eIF4G, which is involved in translation.
Like other ncRNAs, the dysregulation and modification of tsRNAs have been implicated in a significant number of diseases and cancers. It has also been suggested that they may play a major role in autoimmune disorders, with one study finding that 355 tsRNAs are differentially regulated in patients with systemic lupus erythematosus (SLE).13
Long non-coding RNA (lncRNA)
In the genomes of complex organisms, long non-coding RNA (lncRNA) comprises the largest fraction of non-coding RNA. Some cases of lncRNA had been discovered before the year 2000, but their prevalence remained unclear until large-scale transcriptomic studies revealed that most of the human genome was transcribed into lncRNA.
Using an array of research methods, it has been estimated that the human transcriptome contains at least 100,000 lncRNAs. The number of lncRNAs has also been shown to increase with organismal complexity, despite most eukaryotic organisms possessing a similar number of protein-coding genes. For example, both C. elegans and humans have approximately 20,000 protein-coding genes.
It was historically posited that lncRNAs were transcription by-products, but widespread research into dynamic expression has revealed many biological functions for numerous lncRNAs.14
The term lncRNA incorporates a diverse array of RNA molecules featuring various biogenesis pathways, subcellular localizations, and modes of action.
For example, lncRNAs can be intergenic, antisense, or intronic, or they can be derived from pseudogenes. Like mRNA, the majority of lncRNAs are polyadenylated and transcribed by RNA polymerase II.
Loci that produce lncRNAs have many features similar to protein-coding genes, promoters, alternative splicing, multiple exons, chromatin signatures, regulation by morphogens, and conventional transcription factors. They also exhibit altered expression patterns observed in cancer and other diseases.
lncRNAs also include circular RNAs (circRNAs), which are formed through the back-splicing of both coding and non-coding transcripts. This adds to their structural diversity.
Their multiple structured domains can interact with nucleic acids and proteins, meaning that lncRNAs have a diversity of functions. They function through four main mechanisms: acting as signals, acting as decoys for other molecules, guiding ribonucleoprotein complexes to specific targets, and scaffolding larger regulatory complexes.
These mechanisms mean that lncRNAs can impact biological functions at different levels, including epigenetic, transcription, post-transcriptional regulation, genomic stability, and translational.
lncRNAs in the nucleus collaborate in chromatin looping and modification, chromatin remodeling, and control of transcription and splicing. They also function as molecular sponges or decoys by binding other proteins or RNAs. The term competitive endogenous RNA (ceRNA) has been coined to describe this action, as lncRNAs compete with miRNAs, thereby de-repressing the target mRNA.
Enhancer RNA (eRNA) represents a further subclass of lncRNA, transcribed from the DNA sequence of enhancer regions.
Recent research has suggested that eRNAs play an active role in both cis and trans transcriptional regulation, typically interacting with a significant number of proteins. For example, the eRNA from the enhancer that interacts with the promoter of the prostate-specific antigen (PSA) gene has been well studied.
The PSA eRNA is strongly upregulated by the androgen receptor and directly interacts with transcription machinery by binding to and activating the positive transcription elongation factor P-TEFb protein complex.
This process has a domino effect, increasing the expression of approximately 590 androgen receptor-responsive genes. The PSA eRNA could be a novel target that, when suppressed, enhances therapy and diagnosis for prostate cancer.15
lncRNAs are typically expressed in distinct cell types, featuring specific expression patterns unique to each stage of differentiation and development. Around 80 % of lncRNAs exhibit tissue-specific expression, versus 20 % of mRNAs—a figure indicating that these are closely linked to cell fate and developmental trajectory.
The brain includes the most varied collection of lncRNAs, with approximately 40 % of all annotated tissue-specific lncRNAs found in distinct brain regions.
These lncRNAs function at every stage during central nervous system development and homeostasis. Developments in this field have shown that specific lncRNAs contribute to a number of brain disorders, including cancer, Alzheimer’s disease, Parkinson’s disease, and neurodevelopmental disorders.
Discoveries in this field have led to new research into potential therapeutic interventions designed to target these RNAs to restore a typical phenotype.16
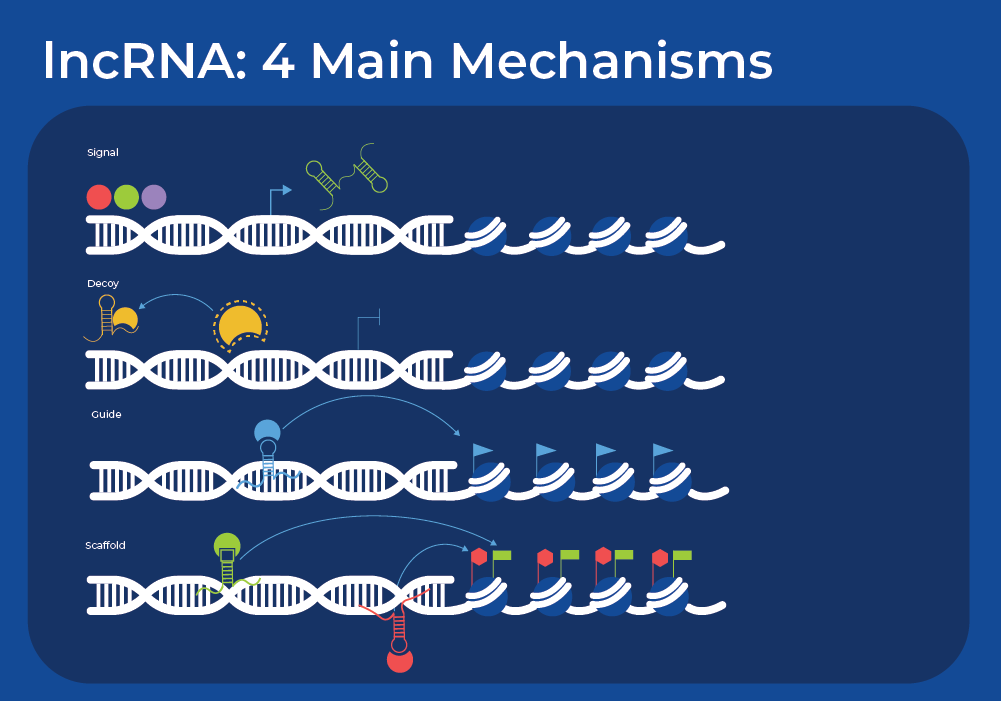
Image Credit: Norgen Biotek Corp.
The epitranscriptome and ncRNA interaction networks
The emerging epitranscriptomic field features a multitude of post-transcriptional modifications with the potential to affect gene expression by impacting RNA localization, stability, folding, and decoding efficiency.
A great deal of this post-transcriptional modification is performed on RNA by other RNAs or RNA-protein complexes. RNAs performing these modifications can be understood as erasers, writers, or readers of modifications.
Beyond the more common roles of snoRNAs and snRNAs in epitranscriptomic modifications, a number of other ncRNAs are either directly or indirectly involved in the epitranscriptome.
Both genetic differences in these modifying RNAs and environmental perturbations affecting them have been implicated in disease, with the epitranscriptome in the parental generation also able to affect organismal phenotypes in the next generation.
RNA regulatory networks can also become more complex due to interactions between various RNA types. This additional layer of ncRNA–ncRNA regulation influences epigenetic modifications, translation, and transcription.
For example, experiments have shown that a circRNA—hsa_circ_0001368—can decelerate gastric cancer growth by regulating miR-6506-5p. It does this by acting as a sponge for the miRNA.
In addition to interactions between different ncRNAs, interactions between the same kinds of ncRNAs also occur. For example, miRNA–miRNA and lncRNA–lncRNA interactions have been observed.
Three distinct miRNAs—miR-125a, miR-125b, and miR-205—have also been shown to act together to suppress erbB2/erbB3 expression in breast cancer cells.
The complete RNA story
Whether studying cancer, host–microbiome interactions, plant development, or gene therapy, researchers must consider the full range of transcribed RNA. The different RNA species discussed here perform a variety of functions and exhibit diverse shapes, sizes, and GC content.
An intricate network of ncRNAs, interacting with other ncRNAs, proteins, and nucleic acids, regulates many phenotypes and genes involved in development and disease.
Norgen Biotek offers a comprehensive portfolio of RNA extraction kits capable of isolating total RNA or just small RNA with minimal bias toward GC content or fragment size. These kits can reveal a sample’s true RNA composition.
Norgen also offers specialized kits designed for plant and fungi RNA, plant miRNA, and leukocyte RNA. The company’s Cytoplasmic and Nuclear RNA Purification Kit is ideally suited for studying RNA localization, and a Single Cell RNA Purification Kit is also available.
Norgen Biotek further provides a range of sample collection and preservation methods designed to stabilize the true diversity of RNA in a sample.
References and further reading
- Fine, J.L. and Pearlman, R.E. (2023). On the origin of life: an RNA-focused synthesis and narrative. RNA, p.rna.079598.123. https://doi.org/10.1261/rna.079598.123.
- Pearce, B.K.D., et al. (2017). Origin of the RNA world: The fate of nucleobases in warm little ponds. Proceedings of the National Academy of Sciences of the United States of America, (online) 114(43), pp.11327–11332. https://doi.org/10.1073/pnas.1710339114.
- Huang, Z., et al. (2022). snoRNAs: functions and mechanisms in biological processes, and roles in tumor pathophysiology. Cell Death Discovery, 8(1). https://doi.org/10.1038/s41420-022-01056-8.
- Kufel, J. and Grzechnik, P. (2019). Small Nucleolar RNAs Tell a Different Tale. Trends in Genetics, (online) 35(2), pp.104–117. https://doi.org/10.1016/j.tig.2018.11.005.
- Liu, Y., et al. (2022). SNORD1C maintains stemness and 5-FU resistance by activation of Wnt signaling pathway in colorectal cancer. Cell Death Discovery, 8(1). https://doi.org/10.1038/s41420-022-00996-5.
- Morais, P., Adachi, H. and Yu, Y.-T. (2021). Spliceosomal snRNA Epitranscriptomics. Frontiers in Genetics, 12. https://doi.org/10.3389/fgene.2021.652129.
- Xiao, L., et al. (2022). Disorders and roles of tsRNA, snoRNA, snRNA and piRNA in cancer. Journal of Medical Genetics, 59(7), pp.623–631. https://doi.org/10.1136/jmedgenet-2021-108327.
- LocanaBio. Available at: https://locanabio.com/ (Accessed 2 Apr. 2025).
- Mraz, M., et al. (2014). miR-150 influences B-cell receptor signaling in chronic lymphocytic leukemia by regulating expression of GAB1 and FOXP1. Blood, 124(1), pp.84–95. https://doi.org/10.1182/blood-2013-09-527234.
- Jones, K., et al. (2013). Plasma MicroRNA Are Disease Response Biomarkers in Classical Hodgkin Lymphoma. Clinical Cancer Research, 20(1), pp.253–264. https://doi.org/10.1158/1078-0432.ccr-13-1024.
- Traber, G.M. and Yu, A.-M. (2022). RNAi-Based Therapeutics and Novel RNA Bioengineering Technologies. Journal of Pharmacology and Experimental Therapeutics, 384(1), pp.133–154. https://doi.org/10.1124/jpet.122.001234.
- Cai, A., et al. (2022). PIWI-Interacting RNAs (piRNAs): Promising Applications as Emerging Biomarkers for Digestive System Cancer. Frontiers in Molecular Biosciences, (online) 9, p.848105. https://doi.org/10.3389/fmolb.2022.848105.
- Liu, B., et al. (2021). Deciphering the tRNA-derived small RNAs: origin, development, and future. Cell Death & Disease, (online) 13(1), pp.1–13. https://doi.org/10.1038/s41419-021-04472-3.
- Mattick, J.S., et al. (2023). Long non-coding RNAs: definitions, functions, challenges and recommendations. Nature Reviews Molecular Cell Biology, 24. https://doi.org/10.1038/s41580-022-00566-8.
- Pan, C.-W., et al. (2021). Functional roles of antisense enhancer RNA for promoting prostate cancer progression. Theranostics, 11(4), pp.1780–1794. https://doi.org/10.7150/thno.51931.
- Srinivas, T., et al. (2023). Roles of lncRNAs in brain development and pathogenesis: Emerging therapeutic opportunities. Molecular Therapy. https://doi.org/10.1016/j.ymthe.2023.02.008.
Acknowledgments
Produced from materials originally authored by Norgen Biotek Corporation.
About Norgen Biotek Corp.
Norgen Biotek: Advancing science with best-in-class, scientist-backed innovations
Norgen Biotek is a fully integrated biotechnology company that focuses on providing complete workflows for molecular biology sample preparation and analysis. With a diverse portfolio of over 600 products, the company delivers high-performance, user-friendly, and cost-effective solutions.
Scientifically driven, industry trusted
Norgen kits cover a broad range of applications from collection and preservation to isolation and purification. Our expert R&D team continuously develops cutting-edge technologies that set new industry standards for RNA, DNA, protein, and exosomal isolation, ensuring superior yield, purity, and integrity from even the most challenging sample types.
Unparalleled performance
At the heart of Norgen’s success is its patented Silicon Carbide (SiC) Technology. This proprietary resin exhibits uniform binding affinity for all RNA species, regardless of molecular weight or GC content. This ensures the full diversity of small and microRNA are captured while eliminating the need for phenol extraction. This innovative technology sets Norgen kits apart from others, positioning them as leaders in RNA purification.
Comprehensive solutions for any challenge
Norgen is committed to providing high-quality kits capable of processing a wide range of sample types, from ultra-low input samples such as liquid biopsies to highly impure samples like stool or soil. Our sample collection and preservation devices for stool and saliva simplify handling by rendering samples non-infectious by preventing microbial growth and inactivating viruses, while our blood and urine preservation solutions ensure the stability of highly vulnerable cell-free nucleic acids.
To meet varying research demands, we offer multiple isolation methods including, but not limited to high-throughput and automation-ready magnetic bead-based formats. Additionally, our multiple-analyte kits enable the simultaneous purification of RNA, DNA, and proteins, maximizing data extraction from a single sample.
Norgen offers an extensive variety of TaqMan qPCR kits designed for molecular diagnostic use, including lyophilized kits for easy shipping. Library preparation kits for both DNA and RNA samples are also available to support genomic applications. Norgen recently released their EXTRAClean technology, an innovative solution that minimizes background noise while providing high-purity RNA, significantly enhancing NGS performance.
Why choose Norgen?
- Scientist-Driven Innovation – Developed by leading experts in molecular biology
- Proven Quality & Reliability – ISO 9001 and ISO 13485 certified, indicating a commitment to selling high-quality products
- Global Presence – We ship to over 150 countries and have a network of 60+ distributors
- Award Winning – Norgen Biotek Corp. was honored with the 2021 Innovative Leaders Award, and recognized as the 2024 Rapid Star Award Winner in the PCR category.
- Innovative Products – Hold more than thirty issued and pending patents for products presenting solutions for all research & clinical applications
Driven by a mission to accelerate scientific discoveries, Norgen Biotek actively supports researchers by providing educational resources, technical workshops, and application notes. Their NorBlog serves as a hub for the latest scientific discoveries, protocol optimizations, and industry trends.
Explore Norgen Biotek’s innovative solutions today and take your research to the next level.
Sponsored Content Policy: News-Medical.net publishes articles and related content that may be derived from sources where we have existing commercial relationships, provided such content adds value to the core editorial ethos of News-Medical.Net which is to educate and inform site visitors interested in medical research, science, medical devices and treatments.