Drug delivery using nanoparticles
There is much research and development devoted to enhancing drug delivery via the utilization of nanoparticles. Although the definition of nanoparticle has become murky, they are usually defined as being in the size range of 100 nm and lower.
In this size range dynamic light scattering (DLS) is the preferred technique and the Nicomp® is often utilized in this field of research. This article outlines how the Nicomp can be employed to study and control nanoparticles which are utilized for drug delivery
Introduction
Both ASTM E24562 and ISO/TS 276871 define nanoparticles as being in the size range of 100 nm or less, making this the most widely employed classification. Interpretations that are less strict have extended the upper size range for both scientific and other reasons.
Now, numerous nanomaterials that are larger than 100 nm in size are commonly called nanoparticles. The motivations for developing drug products in this size range include better dissolution/bioavailability, circulation time in the system, targeting, and area under the curve (AUC) pharmacokinetics.
A number of these drug products are developed in order to improve targeting. By decreasing the size and cloaking the nanoparticle with a coating such as polyethylene glycol (PEG), a passive targeting approach increases the circulation time.
An active targeting approach changes the surface of the nanoparticle to find and adhere to specific parts of the body, like cancer tumors, while avoiding healthy tissue.
In order to bind specifically to complementary receptors, cell-specific ligands on the surface of the nanoparticle can be added. As seen in Figure 1, the Nicomp DLS system is the perfect instrument to measure both the size and zeta potential (surface charge) of nanoparticles employed for drug delivery.
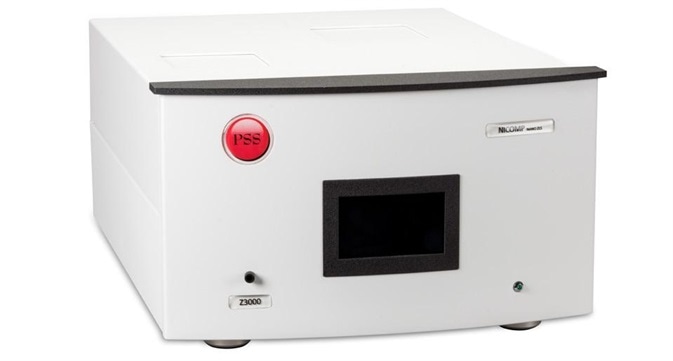
Figure 1. Nicomp DLS instrument. Image Credit: Entegris Particle Sizing
Varieties of nanoparticles
Nanocrystals
Active pharmaceutical ingredients (APIs) are usually crystalline. Hydrophobic crystals can be challenging to formulate to be delivered in a hydrophilic carrier mechanism. A nanosuspension can enhance the bioavailability of drugs by decreasing the size to the nanocrystal range, where the dissolution velocity is the rate-limiting step, like in poorly water soluble drugs.3
These nanocrystals usually require stabilization by utilizing polymers or surfactants, including during processing. A reduction in particle size heightens the dissolution rate by both increasing the saturation solubility Cs and the surface area A (Figure 2).
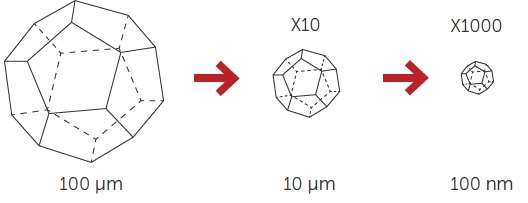
Figure 2. Surface enlargement with size reduction. Image Credit: Entegris Particle Sizing
The Noyes-Whitney equation (Equation 1) demonstrates how an increase in both A and Cs will affect the dissolution rate dC/dt.
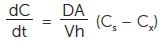 |
(Equation 1) |
Where
dC/dt = dissolution rate
D = diffusion coefficient
A = surface area
Cs = concentration at boundary layer
Cx = concentration API @ given time
V = volume dissolution medium
h = height of boundary layer
Another delivery system that can increase the bioavailability of both hydrophobic and hydrophilic drugs is lipid-based liquid crystalline nanoparticles (LCNPs). These are self-assembled structures which are prepared by the high shear energy dispersing of a nonlamellar liquid crystalline matrix into the water phase.
The particle size of the LCNPs is a vital physicochemical property which needs proper control and analysis. The Nicomp DLS system has been utilized successfully to establish both the mean size and presence of aggregates in LCNP dispersions.4
As seen in Figure 3, paclitaxel was loaded into an LCNP dispersion and analyzed using the Nicomp DLS system and TEM. The TEM image shows a bimodal particle size distribution of smaller near, 25 nm particles, in addition to larger particles on the scale of 100 nm.
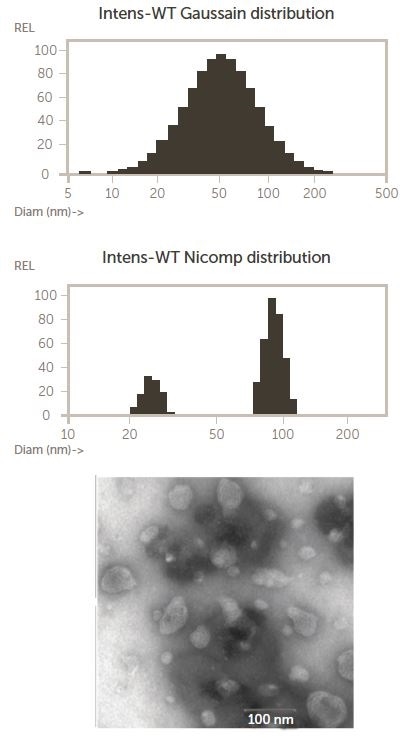
Figure 3. Nicomp and TEM results for an LCNP dispersion, copied with rights from 4. Image Credit: Entegris Particle Sizing
The Gaussian intensity distribution mean is the upper Nicomp result, forcing the entire distribution into one peak. The proprietary Nicomp non-negative least squares algorithm is used by the lower Nicomp result to report a more accurate, higher resolution description of the bimodal nature of the actual particle size distribution.
This shows a key benefit of the Nicomp DLS system – the ability to resolve multi-modal distributions even at concentrations that are as small as 0.2 mg/mL.5
Micelles
Polymeric micelles are another possible drug delivery system that can be used to increase the solubilization of hydrophobic drugs.6 When the concentration of the polymer in solution is higher than a certain threshold concentration known as the critical micellar concentration (CMC), micelles are formed.
Synthesized from amphiphilic block copolymers, polymeric micelles are core-shell nanostructures. Micelles have the benefit of being extremely small in size (10 – 100 nm), enhancing passive targeting to solid tumors. Polymeric micelles can become capable of site-specific drug delivery by altering the surface with ligands.
A number of micelle based research projects have utilized the Nicomp DLS system for particle size measurements.7-11 12 polymeric micelles were formed using copolymers polycaprolactone (PCL) and polyethylene glycol (PEG) in one study.
Docetaxel (DTX) was employed as the model drug and the surface was altered using a small molecular ligand of prostate specific membrane antigen (SMLP). The endocytosis process of the drug loaded final structure and the self-assembly of the micelles is demonstrated in Figure 4.
.jpg)
Figure 4. Preparation and endocytosis of DTX loaded polymeric micelles targeted to PSMA12. Image Credit: Entegris Particle Sizing
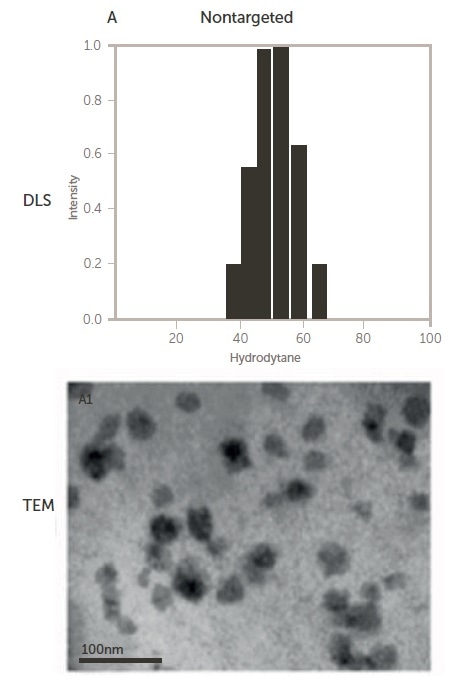
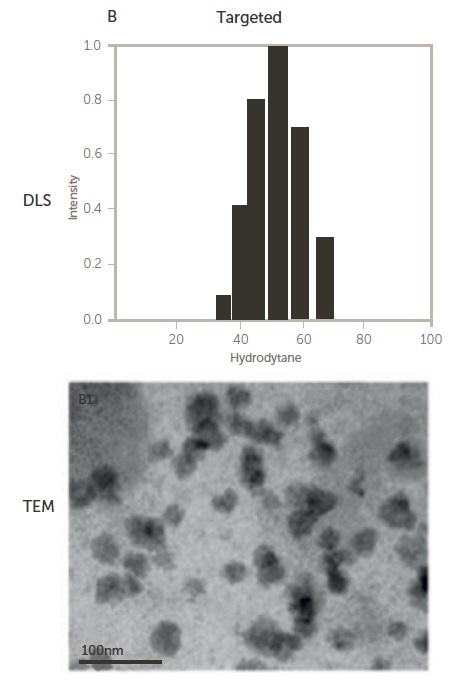
Figure 5. Size of non-targeted (upper) and targeted (lower) polymeric micelles by DLS and TEM 12. Image Credit: Entegris Particle Sizing
Liposomes
Liposomes are bilayer vesicles that are often utilized in the pharmaceutical industry as a drug delivery system for transport of chemotherapeutic drugs to the tumor area.
They are made up of phospholipids, which possess a polar end attached to a nonpolar chain that self-assemble into bilayer vesicles with the nonpolar ends forming a bilayer and the polar ends facing the aqueous medium.
As seen in Figure 6, for pharmaceutical applications, depending on the hydrophilicity of the API, the active pharmaceutical ingredient (API) is typically incorporated into the liposome either into the hydrophilic pocket or sandwiched between the bilayers. For targeted delivery, surface modification is common.
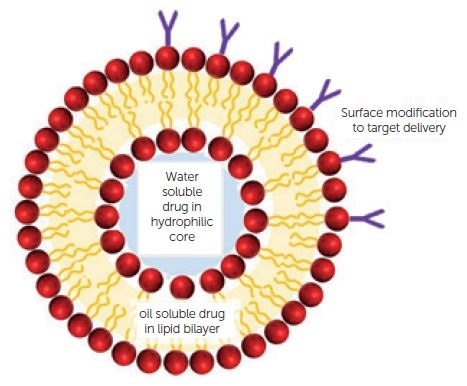
Figure 6. Complex liposome structure. Image Credit: Entegris Particle Sizing
Monitoring the particle size while processing liposomes is crucial and the Nicomp DLS system is often employed for this application.13-20 Liposomes were created using a formulation of 3:1:1 HSPC, cholesterol and mPEG-DSPE in one internal Entegris study.
First, the sample was mixed by a rotor stator at 7200 rpm for 10 minutes. Next, it was passed through a Microfluidizer21 at 25,000 psi utilizing a Y chamber to produce the liposomes. The samples were processed 1, 3, 5, and 10 passes through the microfluidizer.
Figure 7 shows an image of the premix and processed samples from left to right. The liposome samples were analyzed on both the AccuSizer® single particle optical sizing (SPOS) system and the Nicomp DLS system.
-1.jpg)
Figure 7. Pre-mix, 1, 3, 5, and 10 passes. Image Credit: Entegris Particle Sizing
The AccuSizer (LE sensor range 0.5 – 400 μm) was employed to quantify the presence of larger particle tails of the distribution, while DLS was employed to establish the reduction of the intensity mean size during processing. Figure 8 shows the Nicomp DLS results and the AccuSizer SPOS results can be seen in Figure 9.
.jpg)
Figure 8. Nicomp DLS results from right to left; premix, 1, 3, 5, and 10. Image Credit: Entegris Particle Sizing
.jpg)
Figure 9. AccuSizer SPOS results right to left; premix, 1, 3, 5, and 10 passes. Image Credit: Entegris Particle Sizing
In a number of industries, using both DLS to establish the mean size and SPOS to quantify the presence and concentration of tails is common and is an integral part of USP <729> Globule-size distribution in lipid injectable emulsions.22
Online Dls for process monitoring
Whilst most DLS measurements are performed in the laboratory, Entegris has installed numerous systems in customer manufacturing operations that track particle size during production runs.23
These systems have been utilized to monitor the high-pressure homogenization processes which are employed during the manufacture of nanoparticles for drug delivery. As seen in Figure 10, the at-line system takes away a sample from the process, dilutes it to avoid multiple scattering effects, measures it, and then repeats the procedure.
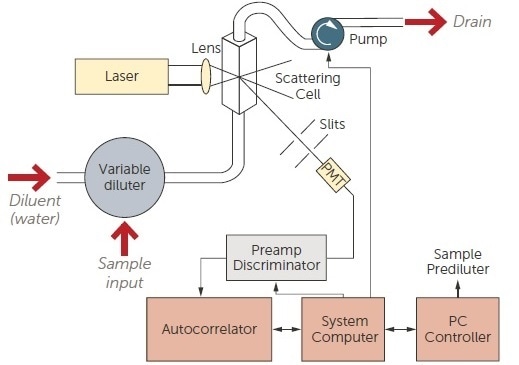
Figure 10. Online DLS system schematic. Image Credit: Entegris Particle Sizing
The complete measurement cycle takes around two minutes and supplies continuous particle size information to the process engineers who are monitoring the manufacturing operation. Online DLS results as a function of pressure downstream of a high pressure homogenizer can be seen in Figure 11.
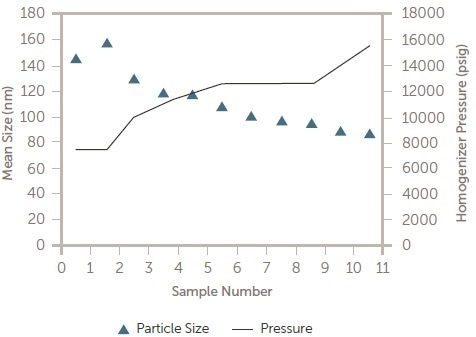
Figure 11. Pressure vs. particle size in process DLS results. Image Credit: Entegris Particle Sizing
The aim of this was to establish the optimum pressure to keep the particle size very near to 100 nm in size. After the optimum pressure (~10 k psi) was established, the online DLS system was employed to ensure the complete batch was manufactured within specification.
Conclusions
The Nicomp DLS system is widely utilized for particle size and zeta potential analysis of drug delivery systems in the nano scale in research,24-39 quality release testing and in process monitoring.
The AccuSizer SPOS supplies a complementary method for establishing the concentration of larger particles, which could show instability or non-optimized formulation or process conditions.
References and further reading
- ISO/TS 27687, Nanotechnologies—Terminology and definitions for nanoobjects—Nanoparticle, nanofibre and nanoplate, available at https://www.iso.org/obp/ui/#iso:std:iso:ts:27687:ed-1:v2:en
- ASTM E2456, Standard Terminology Relating to Nanotechnology, available at Standards/E2456.htm
- Jens-Uwe et al., Nanocrystal technology, drug delivery and clinical applications, International Journal of Nanomedicine 2008:3(3) 295–309
- Zeng et al., Lipid-based liquid crystalline nanoparticles as oral drug delivery vehicles for poorly water-soluble drugs International Journal of Nanomedicine 2012:7
- Scomparin et al., Novel folated and non-folated pullulan bioconjugates for anticancer drug delivery European Journal of Pharmaceutical Sciences 42 (2011) 547–558
- Cory et. Al, Polymeric Micelles for Drug Delivery, Curr Pharm Des. 2006;12(36):4669-84
- Koizumi et al., Novel SN 38 Incorporating Polymeric Micelles, NK012 Eradicate Vascular Endothelial Growth Factor Secreting Bulky Tumors, Cancer Res 2006; 66: (20) with Nicomp data
- Song et al., Self-assembled micelles of novel amphiphilic copolymer cholesterol-coupled F68 containing cabazitaxel as a drug delivery system, Int J Nanomedicine. 2014; 9: 2307–2317.
- Wang, Pharmacokinetics and Biodistribution of Paclitaxel-loaded Pluronic P105/L101 Mixed Polymeric Micelles, Pharmaceutical Society of Japan, 128(6), 2008
- Bachar et al., Development and characterization of a novel drug nanocarrier for oral delivery, based on self-assembled b-casein micelles, Journal of Controlled Release, Volume 160, Issue 2, 10 June 2012
- Jiang et al., Poly(aspartic acid) derivatives as polymeric micelle drug delivery systems J Appl Polym Sci 101: 2871–2878, 2006
- Jin et al., PSMA Ligand Conjugated PCL-PEG Polymeric Micelles Targeted to Prostate Cancer Cells, PLoS ONE 9(11): e112200. doi:10.1371/journal.pone.0112200
- Zidan et al., Near-Infrared Investigations of Novel Anti-HIV Tenofovir Liposomes, The AAPS Journal, Vol. 12, No. 2, June 2010
- Wong et al., A New Polymer-Lipid Hybrid Nanoparticle System Increases Cytotoxicity of Doxorubicin Against Multidrug-Resistant Human Breast Cancer Cells, Pharmaceutical Research, Vol. 23, No. 7, July 2006
- Zhang et al., The cargo of CRPPR-conjugated liposomes crosses the intact murine cardiac endothelium, J Control Release, 2012 October 10; 163(1)
- Guan et al., Enhanced oral bioavailability of cyclosporine A by liposomes containing a bile salt, International Journal of Nanomedicine 2011:6
- Ando et al., Reactivity of IgM antibodies elicited by PEGylated liposomes or PEGylated lipoplexes against auto and foreign antigens, Journal of Controlled Release, Volume 270, 28 January 2018
- Johnston et al., Characterization of the drug retention and pharmacokinetic properties of liposomal nanoparticles containing dihydrosphingomyelin, Biochimica et Biophysica Acta 1768 (2007)
- Cipolla et al., Modifying the Release Properties of Liposomes Toward Personalized Medicine, Journal of Pharmaceutical Sciences 103:1851–1862, 2014
- El-Ridy et al., Liposomal Encapsulation of Amikacin Sulphate for Optimizing Its Efficacy and Safety, BJPR, 5(2): 98-116, 2015
- Entegris Application Note Size Reduction by a Microfluidizer,
- Entegris Application Note USP 729 Testing
- Entegris Application Note Nanoparticles for Drug Delivery
- Wong et al., A New Polymer-Lipid Hybrid Nanoparticle System Increases Cytotoxicity of Doxorubicin Against Multidrug-Resistant Human Breast Cancer Cells, Pharmaceutical Research, Vol. 23, No. 7, July 2006
- Martins et al., Brain delivery of camptothecin by means of solid lipid nanoparticles: Formulation design, in vitro and in vivo studies, International Journal of Pharmaceutics 439 (2012) 49– 62
- Podaralla et al., Influence of Formulation Factors on the Preparation of Zein Nanoparticles, AAPS PharmSciTech, Vol. 13, No. 3, September 2012
- Chertok et al., Iron oxide nanoparticles as a drug delivery vehicle for MRI monitored magnetic targeting of brain tumors, Biomaterials, Volume 29, Issue 4, February 2008
- Songa et al., Formulation and characterization of biodegradable nanoparticles for intravascular local drug delivery, Journal of Controlled Release, Volume 43, Issues 2–3, 18 January 1997
- Jain et al., Magnetic nanoparticles with dual functional properties: Drug delivery and magnetic resonance imaging, Biomaterials, Volume 29, Issue 29, October 2008
- Guo et al., Aptamer-functionalized PEG–PLGA na-noparticles for enhanced anti-glioma drug delivery, BiomaterialsVolume 32, Issue 31, November 2011
- Nguone et al., Accumulating nanoparticles by EPR: A route of no return, Journal of Controlled Release Volume 238, 28 September 2016Menzel et al., In vivo evaluation of an oral self-emulsifying drug delivery system (SEDDS) for exenatide, Journal of Controlled Release, Volume 277, 10 May 2018
- Dorati et al., Gentamicin Sulfate PEG-PLGA/PLGA-H Nanoparticles: Screening Design and Antimicrobial Effect Evaluation toward Clinic Bacterial Isolates, Nanomaterials 2018, 8, 37
- Xu et al., The performance of docetaxel-loaded solid lipid nanoparticles targeted to hepatocellular carcinoma, Biomaterials 30 (2009) 226–232
- Piao et al., Human serum albumin-coated lipid nano-particles for delivery of siRNA to breast cancer, Na-nomedicine: Nanotechnology, Biology, and Medicine 9 (2013)
- Andersen et al., Chitosan-Based Nanomedicine to Fight Genital Candida Infections: Chitosomes, Mar. Drugs 2017, 15, 64
- Kou et al., Preparation and characterization of the Adriamycinloaded amphiphilic chitosan nanoparti-cles and their application in the treatment of liver cancer, Oncology Letters 17: 7833-7841, 2017
- Kuang et al., Dual Functional Peptide-Driven Nano-particles for Highly Efficient Glioma-Targeting and Drug Codelivery, Molecular Pharmaceutics, April, 2016
- Cooper et al., Formulation and in vitro evaluation of niacin-loaded nanoparticles to reduce prostaglandin mediated vasodilatory flushing, European Review for Medical and Pharmacological Sciences, 2015; 19: 3977-3988
- Martins et al., Physiochemical properties of lipid na-noparticles: Effect of lipid and surfactant composition, Drug Development and Industrial Pharmacy 37(7):815-24
About Entegris Particle Sizing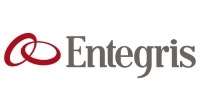
Since our inception in 1978 we have been providing leading edge instrumentation for the field of particle size analysis. We are an applications driven company that provides solutions to our customer's most complex particle sizing and zeta potential monitoring problems. From wet to dry, online to research laboratory we have engineered a complete family of modular designed instruments that can be configured to meet the specific demands of an application.
Single particle optical sizing (SPOS)
The Entegris AccuSizer operates using the principle of single particle optical sizing (SPOS). This technique generates high accuracy, high resolution particle size distribution results as well as concentration data in particles/mL. The AccuSizer platform includes multiple configurations designed to meet a wide array of applications including laboratory and online systems. Multiple sensor options cover a wide range of particle size and concentration limits. Advanced fluidics modules can handle low concentration samples requiring no dilution to highly concentrated inks and CMP slurries using our patented two stage exponential dilution system.
Dynamic light scattering
The Nicomp dynamic light scattering (DLS) system measures particle size below 1 nm and zeta potential to study particle charge. The unique Nicomp algorithm provides the highest resolution results for samples containing more than one peak. Other unique capabilities include auto-dilution and auto-samplers to facilitate high sample throughput.
Sponsored Content Policy: News-Medical.net publishes articles and related content that may be derived from sources where we have existing commercial relationships, provided such content adds value to the core editorial ethos of News-Medical.Net which is to educate and inform site visitors interested in medical research, science, medical devices and treatments.