Sponsored Content by MaxCyte, Inc.Reviewed by Maria OsipovaOct 19 2023
Oligonucleotide drugs are an emerging area of development that hold great promise for personalized treatment of rare diseases.
There are approximately 8,000 distinct rare diseases, each individually affecting fewer than one in 2,000 people. Collectively, rare diseases impact more than 300 million people globally.
Many of these diseases have a genetic factor that makes them excellent targets for personalized oligonucleotide therapies based on short sequences of nucleic acids. These are designed to regulate protein and mRNA expression to address diseases at the transcriptome level.
Friedrich’s Ataxia (FRDA) is a rare genetic disease produced by a mutation in the frataxin (FXN) gene, which codes for a mitochondrial protein. In patients with FRDA, a trinucleotide repeat expansion in non-coding DNA results in the associated mRNA creating an R-loop, impeding transcription of FXN. No therapies address FRDA at the transcriptome level via the up-regulation of FXN expression.
All oligonucleotide drugs function by base-pairing with target sequences in the affected gene. For FRDA, the target sequence is the FXN repeat expansion.
Two types of oligonucleotide drugs exist: RNA interference (RNAi) and antisense oligonucleotides (ASOs) therapies. ASOs operate as a single strand and frequently carry chemical modifications to improve their stability and binding affinity with the target.
RNAi drugs are based on small interfering RNA (siRNA) that operate with the cells’ RNA silencing machinery to modify gene expression.
Researchers have previously shown that siRNAs and ASOs may efficiently target the FXN repeat expansion and improve FXN protein expression in FRDA patient-derived fibroblasts.
MaxCyte® Flow Electroporation technology is now assisting these researchers to investigate the efficacy of oligonucleotide therapies in induced pluripotent stem cell-derived neural progenitor cells (iPSC-NPCs) from FRDA patients.
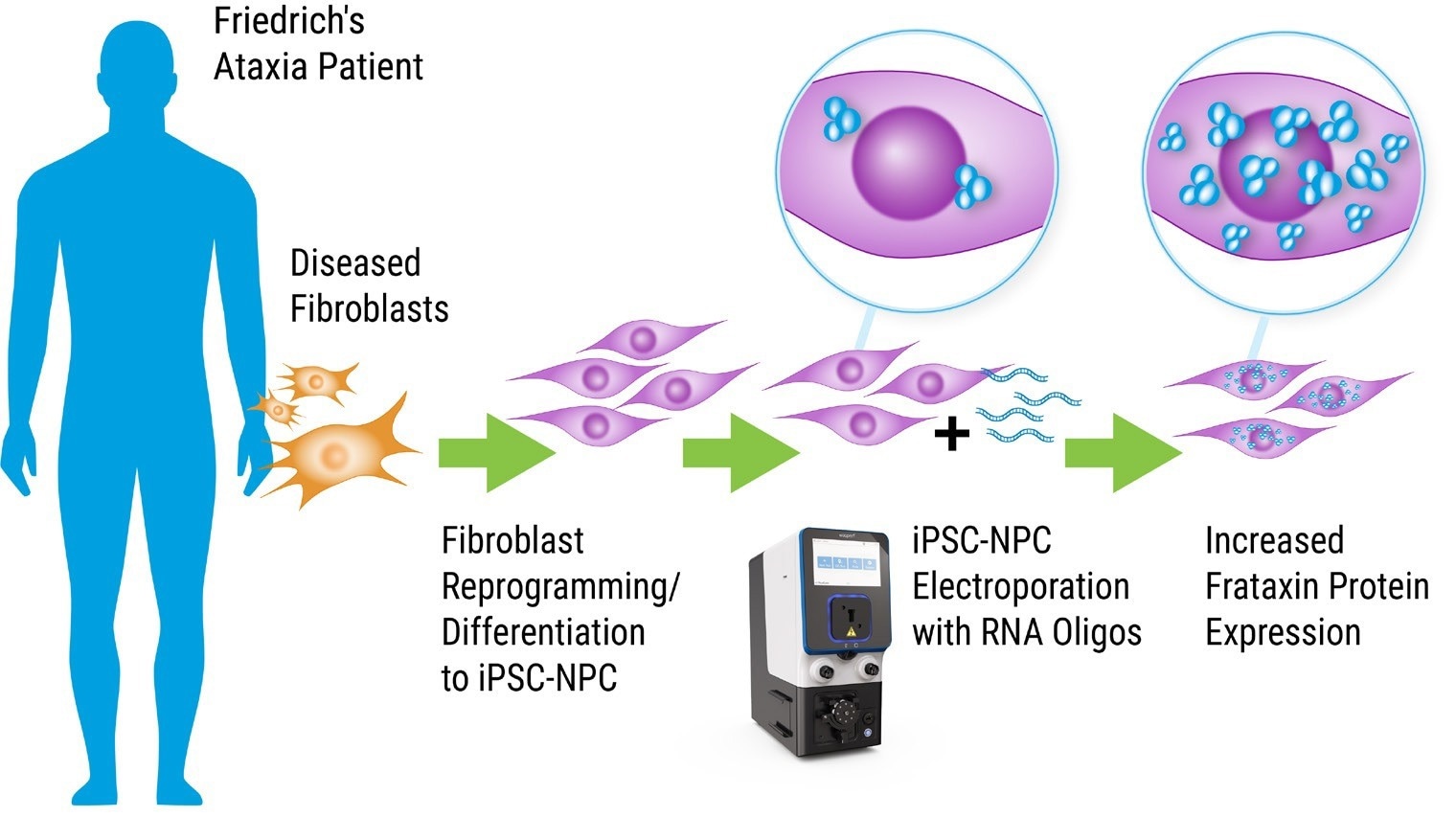
MaxCyte Flow Electroporation®: A Practical Method for Oligonucleotide Drug Discovery. Image Credit: Adapted from Shen et al. 2019 doi: 10.1261/rna.071290.119 under Creative Commons license Attribution 4.0 International (CC BY 4.0)
Aim
The study detailed in this article explored oligonucleotide activators as candidates for FRDA treatment.1
The researchers initially aimed to identify an efficient and reproducible approach for delivering synthetic oligonucleotide drug candidates into patient-derived iPSCs. An ideal solution for this was MaxCyte’s Flow Electroporation® technology.
After introducing the oligonucleotides through highly efficient and safe ExPERT™ electroporation, featured experiments explored their proficiency in up-regulating FXN protein and mRNA expression.
Method
Below is a summary of the protocol used to reprogrammed and differentiated patient-derived iPSCs then transfected oligonucleotides into the resultant iPSC-NPCs to evaluate their impact on gene expression.
The method demonstrates how well-suited MaxCyte Flow Electroporation is to developing and delivering novel gene therapies.
1. Reprogramming of fibroblast cell lines to iPSCs was conducted utilizing integration-free Sendai virus transgene delivery.
2. Differentiation of the iPSCs into NPCs by inhibition of TGF-β/SMAD signaling.
3. Transfection of iPSC-NPCs utilizing the MaxCyte STx™ instrument with preset Optimization protocols 4 and 6 and OC-100 processing assembly to produce synthetic siRNA and ASOs drug candidates. Following transfection, cells briefly recovered at 37 °C and were transferred to 12- or 24-well plates.
4. Analysis of FXN protein and mRNA expression after being monitored by qRT-PCR and then western blot 24-96 hours post-transfection.
Results
Efficient electroporation and transcript knockdown
A previously recognized target gene, benchmark oligonucleotide compound (MALAT1 and anti-MALAT1), and human motor neuron cells (HMN, iXCells Biotechnologies) were employed for the primary assessment of transfection techniques.
After either Lipofectamine® stem transfer reagent, MaxCyte electroporation, or gymnotic (unassisted) uptake, post transfection cell viability and knockdown of MALAT1 24 h were compared for each method.
A 1 µM of the anti-MALAT1 ASO delivered by MaxCyte electroporation gave over 95% RNA knockdown in NPCs and HMNs, as displayed in Figures 1A and 1C. This is substantially better compared to gymnotic delivery or lipid transfection.
Cell viability post electroporation was demonstrated as equivalent or superior to cells that received oligo alone via gymnotic free uptake or lipid transfection, as shown in Figures 1B and 1D.
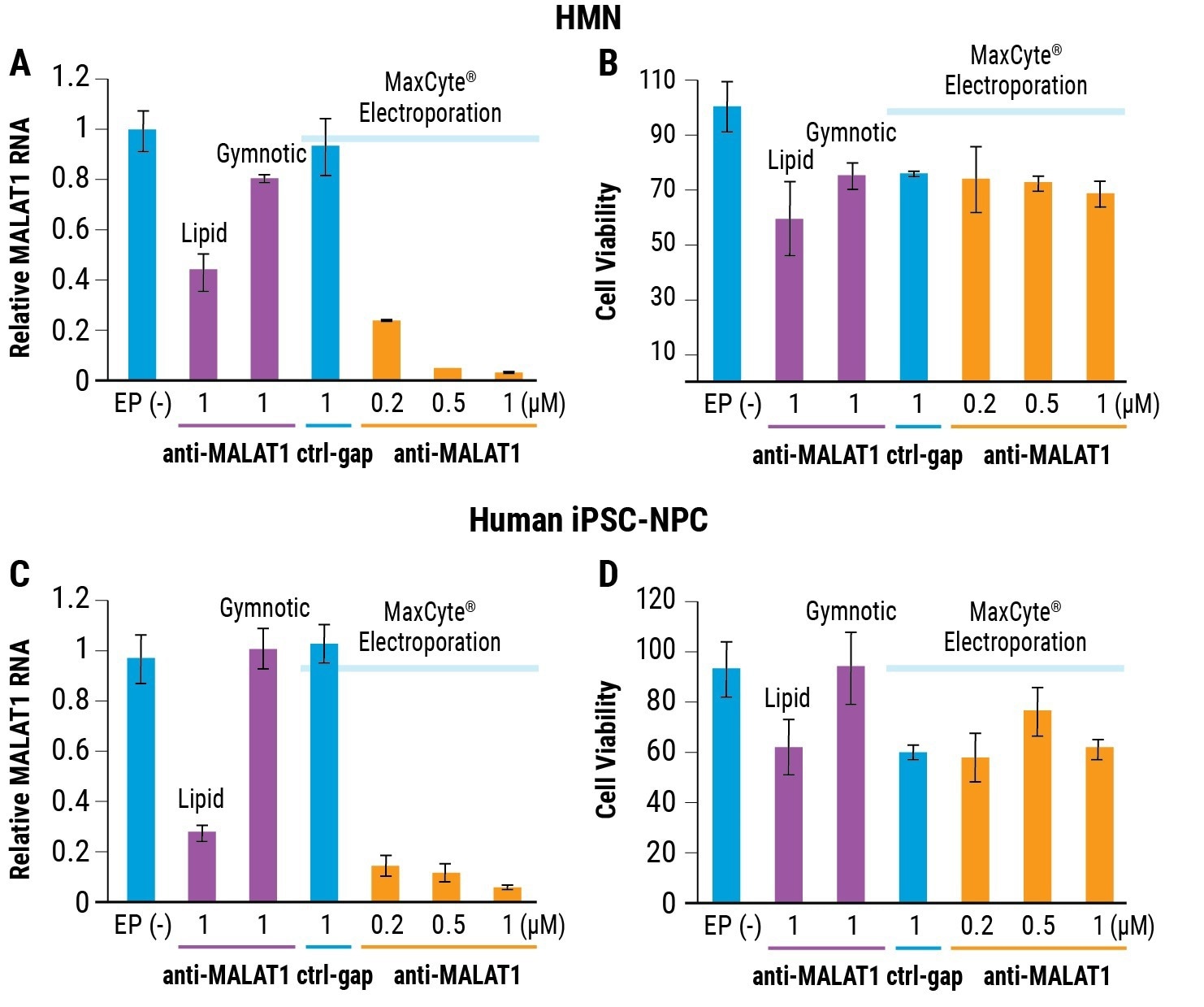
Figure 1. Electroporation for optimized cell viability and RNAi efficiency. A) Relative MALAT1 RNA levels (measured by RT-qPCR) and B) relative HMN cell viability (measured by Trypan blue staining) after transfection by either lipid transfection, gymnotic delivery or MaxCyte electroporation. C) Relative MALAT1 RNA levels in FRDA patient-derived iPSC-NPCs after transfection by MaxCyte electroporation. D) Relative cell viability was measured by Trypan blue staining. All assays performed 24h post transfection with anti-MALAT1. EP(-) is no treatment/no electroporation control. Ctrl-gap is a control oligonucleotide. Error bars represent ± SD. Image Credit: Adapted from Shen et al. 2019 doi: 10.1261/rna.071290.119 under Creative Commons license Attribution 4.0 International (CC BY 4.0)
Up-regulated frataxin expression
The expression of FXN mRNA in FRDA patient-derived iPSC-NPCs was evaluated with and without three distinct oligonucleotide activators:
- BNA-2 is an ASO containing a constrained (bridged) ethyl modification on the ribose for improved binding affinity to the repeat expansion
- ss-siRNA-1 is a single-stranded siRNA that utilizes the RNAi mechanism
- A control named ctrl-ASO
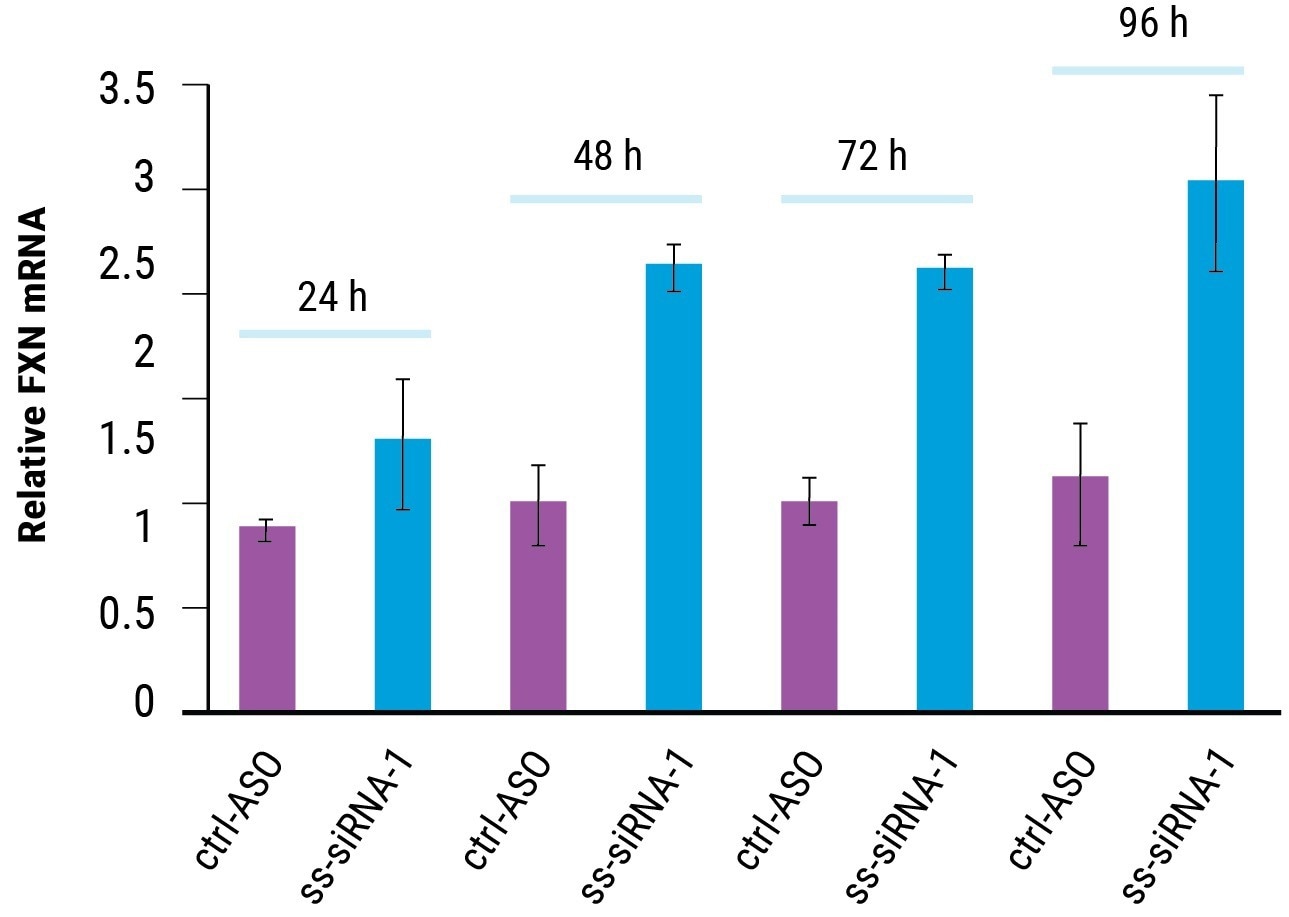
Figure 2. Time course of FRDA activation. fxn mRNA expression in FRDA patient-derived iPSC-NPCs with ss-siRNA-1 (5 μM, n = 2, optimization 4) and control oligonucleotide ctrl-ASO. Error bars represent ± SD. Image Credit: Adapted from Shen et al. 2019 doi: 10.1261/rna.071290.119 under Creative Commons license Attribution 4.0 International (CC BY 4.0)
FXN mRNA was expressed in iPSC-NPCs from a healthy person 2.5 times more than that of FRDA patients.
MaxCyte electroporation of 5 µM ss-siRNA (as displayed in Figure 2) or BNA-2 (data not shown) elevated FXN expression in FRDA patient-derived iPSC-NPCs to normal levels within 48 hours. FXN mRNA continued to be elevated until at least 96 hours post transfection.
As predicted, the activation of FXN mRNA expression led to increased protein expression. Delivered at 5 µM by MaxCyte Flow Electroporation, BNA-2 and ss-siRNA-1 increased frataxin protein threefold, near the level expressed in healthy cells (as shown in Figure 3).
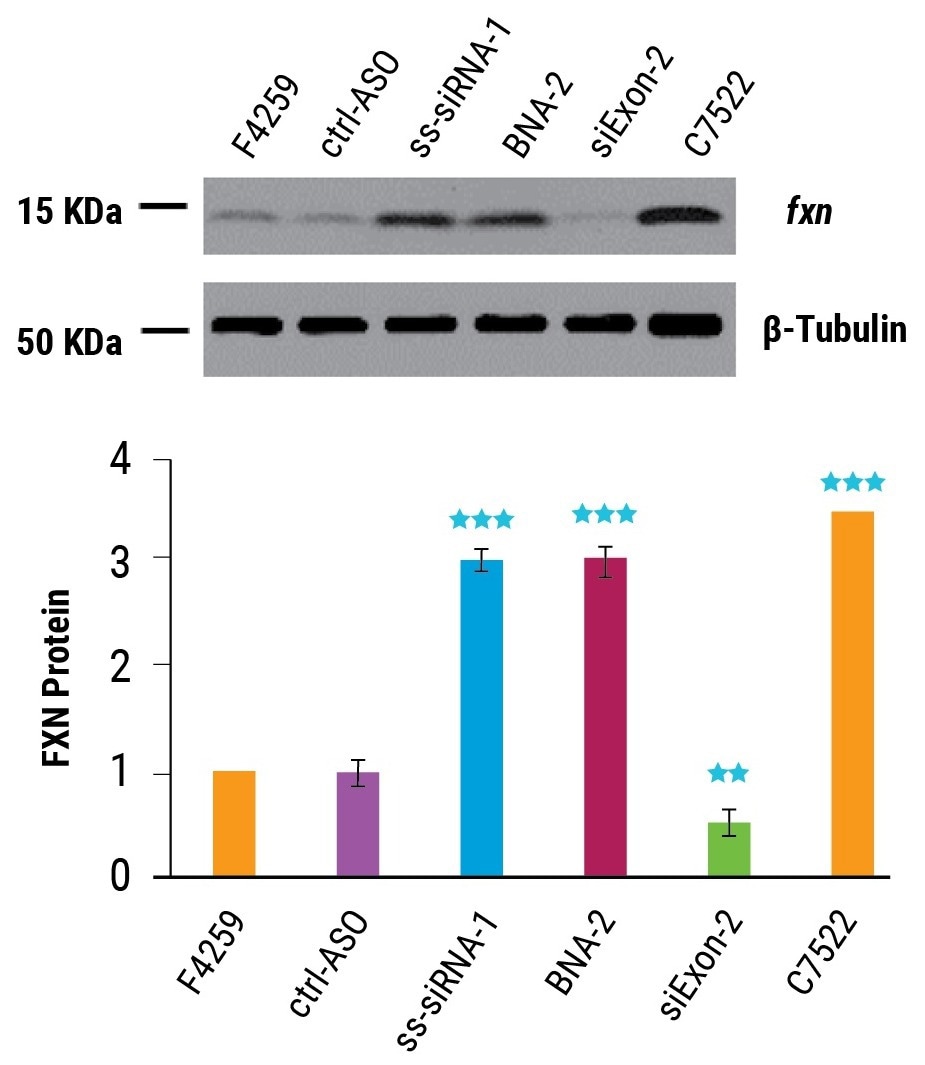
Figure 3. Activation of Frataxin protein expression. FRDA patient-derived NPCs (F4259) and wild-type NPCs (C7522) were electroporated using the MaxCyte STx™ with oligonucleotides (5 μM, n = 3) and FXN protein measured 96 hr post transfection by western blot. Error bars represent ±SD. ** indicates P < 0.01 and *** indicates P < 0.001 relative to F4259-90% by student t-test. Image Credit: Adapted from Shen et al. 2019 doi: 10.1261/rna.071290.119 under Creative Commons license Attribution 4.0 International (CC BY 4.0)
Enhanced potency through backbone modification
MaxCyte Flow Electroporation facilitated the assessment of a matrix of ASOs and siRNAs with distinct backbone modifications (bridged ethyl and 2’-methoxyethyl) to determine the efficacy of each potential drug regarding the up-regulation of FXN mRNA.
Measurements of FXN expression were taken at several doses and half maximal effective concentration (EC50) was determined for each oligonucleotide, as shown in Table 1. The most potent (lower EC50) drugs were constrained ethyl ASOs and siRNAs.
Table 1. Synthetic oligonucleotide effect on fxn mRNA expression in FRDA patient-derived iPSC-NPCs. cET = constrained ethyl, 2’-O-MOE = 2’-methoxyethyl. The most potent drug from each type of backbone chemistry is highlighted. Source: Adapted from Shen et al. 2019 doi: 10.1261/rna.071290.119 under Creative Commons license Attribution 4.0 International (CC BY 4.0)
Oligonucleotide |
Type |
Backbone Chemistry |
Length
(bases) |
EC50 (μM) |
ss-siRNA-1 |
siRNA |
ssRNA |
21 |
0.62 ± 0.19 |
siGAA |
siRNA |
dsRNA |
21 |
0.80 ± 0.44 |
BNA-2 |
ASO |
cET |
16 |
0.50 ± 0.09 |
BNA-9 |
ASO |
cET |
16 |
0.71 ± 0.19 |
BNA-17 |
ASO |
cET |
18 |
- |
M-1 |
ASO |
2'-O-MOE |
16 |
2.00 ± 0.84 |
M-2 |
ASO |
2'-O-MOE |
16 |
2.18 ± 0.99 |
M-3 |
ASO |
2'-O-MOE |
16 |
2.06 ± 0.68 |
M-4 |
ASO |
2'-O-MOE |
18 |
1.07 ± 0.38 |
M-5 |
ASO |
2'-O-MOE |
18 |
0.75 ± 0.37 |
M-6 |
ASO |
2'-O-MOE |
18 |
1.74 ± 0.63 |
Benefits of MaxCyte Electroporation
This research shows a unique solution for the introduction of synthetic oligonucleotide therapies into iPSC-derived cells. MaxCyte Flow Electroporation was less toxic, more efficient, and more straightforward for inexperienced users to implement to attain successful outcomes, in contrast to lipid transfection.
The researchers expect electroporation to be an invaluable tool for testing novel therapies and facilitating rapid drug development processes.
Conclusion and future applications
MaxCyte technology is assisting research into innovative gene therapies to tackle rare genetic diseases.
The research detailed in this article shows that MaxCyte Flow Electroporation is an effective and reproducible technique for testing panels of oligonucleotide drugs in patient-derived neural progenitor cells that demonstrate great promise for the up-regulation of protein and gene expression to therapeutic levels.
The results presented offer a framework for the use of Flow Electroporation as a valuable tool for the development of oligonucleotide therapies in addition to candidate screening and lead optimization strategies for genetic disorders at the transcriptome level.
References and further reading
- Shen X, Beasley S, Putman JN, et al. RNA. 2019;25(9):1118-1129. doi: 10.1261/rna.071290.119.
- Simic. Croat Med J. 2019 Dec; 60(6): 485–487. doi: 10.3325/cmj.2019.60.485
About MaxCyte, Inc.
MaxCyte is a leading commercial cell-engineering company focused on providing enabling platform technologies to advance innovative cell-based research as well as next-generation cell therapeutic discovery, development and commercialization. Over the past 20 years, we have developed and commercialized our proprietary Flow Electroporation® platform, which facilitates complex engineering of a wide variety of cells.
Our ExPERT™ platform, which is based on our Flow Electroporation technology, has been designed to support the rapidly expanding cell therapy market and can be utilized across the continuum of the high-growth cell therapy sector, from discovery and development through commercialization of next-generation, cell-based medicines. The ExPERT family of products includes: four instruments, the ATx™, STx™, GTx™, and VLx™; a portfolio of proprietary related processing assemblies or disposables; and software protocols, all supported by a robust worldwide intellectual property portfolio.
Sponsored Content Policy: News-Medical.net publishes articles and related content that may be derived from sources where we have existing commercial relationships, provided such content adds value to the core editorial ethos of News-Medical.Net which is to educate and inform site visitors interested in medical research, science, medical devices and treatments.