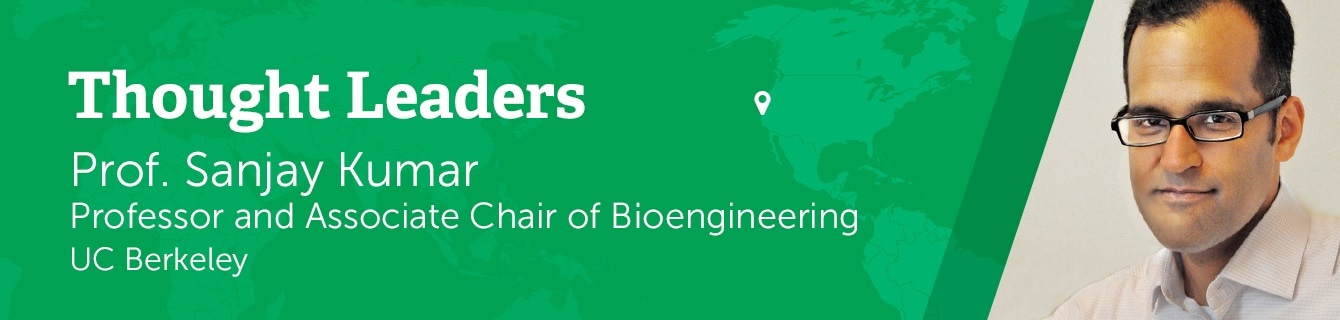
An interview with Prof. Sanjay Kumar, UC Berkeley conducted by April Cashin-Garbutt, MA (Cantab)
Please can you give a brief introduction to your research on cell mechanics and cell function?
My lab, broadly speaking, is interested in trying to understand interactions between cells and materials. Those could be materials that are present within living tissue or materials that we use for engineering purposes to try to do something of technological or therapeutic interest.
Credit: mkarco/Shutterstock.com
A lot of our work builds on a set of observations that have been made in the field over the past couple of decades that the mechanical properties of materials are very important in governing cell behavior in a variety of contexts.
To that end, we are very interested in the fundamental mechanisms through which cells sense, process and respond to mechanical signals, as well as trying to think about ways we can use those signals to engineer cell behavior in various ways.
How important is AFM to your research?
AFM is very important to us because it provides the quantitative means to measure mechanical properties, not just of cells, but also of the biomaterials in which we culture cells.
What is helpful with AFM is that the measurements can be done under physiologic conditions, so to speak, so under aqueous conditions, using the sorts of buffers and media that we would use for living cells and tissues and operating at the mechanical scales that I think are most relevant and appropriate for biological tissues.
They are capable of very sensitive measuring of mechanical properties in the range that we would like to measure them in. We use AFM quite a lot to measure the viscoelastic properties of materials that we culture cells on as well as living cells and tissues. It is one of the few technologies that can make those kinds of measurements using living tissue.
Studying Cell Mechanics with AFM
Studying Cell Mechanics with AFM from AZoNetwork on Vimeo.
What advances have been made in understanding how the mechanical properties of cells play a role in cancer and stem cell differentiation?
In the field of cancer, there has been a very long-standing observation that the progression of tumors is often associated with or correlated with changes in mechanical properties of the tissue. A clinician might tell you that if they palpate a soft tissue and find a stiff nodule, that nodule needs to be investigated. There is a long-standing correlation between the stiffness of a lesion and the severity of disease prognosis.
I think the major advance that has been made over the past 20 to 30 years is the recognition that changes in tissue mechanics is not just something that happens over the course of the disease; cell and tissue mechanics can play very important roles in driving disease progression. It has been discovered that in many cancer types, changes in the stiffness of the surrounding tissue can help to drive tumor progression and this may present an opportunity to develop new therapies that arrest that interaction.
In the case of stem cell engineering, there is a similar, but distinct story, where it has been discovered that the changes in mechanical properties of materials can help to guide stem cells as they make fate decisions to differentiate into specific tissue types, which raises the obvious possibility of engineering materials in order to control stem cell differentiation.
Can you please outline how you have been exploring mechanobiological signalling systems as targets for limiting the invasion of brain tumors and enhancing stem cell neurogenesis?
As I mentioned, in cancer, it's becoming clear in many systems that changes in tissue mechanics can help to guide or influence tumor progression. In the case of brain cancer, especially glioblastoma, which is the tumor that we study most heavily in my lab, the reason that this has such a poor prognosis and ultimately kills patients is that the tumors spread in very aggressive ways within the brain tissue. The way they do that is very specific. Often, tumor cells will home towards very stiff structures within the brain, including blood vessels, and use those as contact guidance cues or, if you like, highways of a sort, to infiltrate tissue.
The thinking is that mechanical differences in blood vessels relative to the surrounding brain tissue could be important in helping to promote or drive the invasion process. Again, it raises this possibility that if we could identify how this recognition process works, we may be able to develop a completely new set of druggable targets to try to arrest the invasion process.
Part of our work involves trying to identify those targets. Another part involves trying to develop new tissue culture systems that simulate the structures within the brain and enable us to do things like drug discovery and screening.
In the case of stem cells, a lot of our most exciting recent work has focused on trying to understand how the signaling systems that regulate mechanical signaling interface with signaling systems that the field normally thinks about as controlling stem cell differentiation.
We have been studying interactions between these various signaling systems and asking how a mechanical force, for example, leads to activation of genes that are traditionally understood to drive stem cell neurogenesis. Investigating that interface has really opened up a lot of very interesting questions for us.
How are you creating new biomaterials inspired by cellular structural networks?
This goes back to some work that I had done many years ago when I was a graduate student. To make a long story short, we recognized that a type of cytoskeletal protein called intermediate filaments within nerve cells, assembles using strategies that are similar to those people use to stabilize paints, colloids and other suspensions that are used industrially.
The basic idea is that these intermediate filaments essentially act as cylindrical particles and those particles are coated by a layer of proteins that helps to prevent two particles from crashing into each other and aggregating.
The coatings themselves are very interesting. They are made up of single protein chains and they can expand or collapse in response to chemical modification, as well as the ionic strength and pH of the medium that surrounds them.
Over the last few years, we have been trying to figure out how to make these protein domains artificially, assemble them as surfaces, use them as coatings for particles and see if we can develop new technologies that are essentially based on a system that nature has given us.
What is the biggest impact that AFM has made to the biological and nanomedicine research fields?
It is hard to identify one single biggest impact, but I can tell you about two or three of them that stand out in my mind.
One is along the lines of what I described, giving us a way to sensitively and precisely characterize mechanical properties of tissues and biomaterials that are relevant to understanding and controlling physiology.
Another major contribution has been in imaging. Atomic force microscopy is capable of going where other techniques cannot in terms of characterizing the dynamics of biomolecules under aqueous conditions, that is, in water, which is where life occurs and, more recently, doing this very quickly. We are able to observe dynamic processes such as molecular motors walking along cytoskeletal filaments in ways that are simply not possible with other imaging technologies.
Finally, AFM has helped us to understand the forces that are involved in controlling the structure within proteins and DNA. There is a very famous set of experiments starting from about 20 years ago where investigators were able to take single molecules such as proteins and use AFM to literally unfold them or change their structure by force. This has added a great deal to our basic understanding of how proteins assemble, fold, and, ultimately, create functional structures.
How has Bruker technology helped or advanced AFM in biological research?
Bruker, in all of its various incarnations as an AFM manufacturer, has been a major player in making AFMs and in supporting atomic force microscopy research, particularly its application in the life sciences, and bringing users together at conferences such as this one, to discuss the cutting edge in the field.
I think one area where Bruker has particularly been at the cutting edge is in integrating atomic force microscopy technology with other technologies that are traditional and useful for a life scientist, especially optical microscopy. That is an area where they have done very well − trying to anticipate the needs of life sciences researchers and figuring out ways to accommodate those needs with AFM technology.
What is the importance of meetings, like the AFM BioMed Conference, to you and the AFM research community?
It is very important for us to have a forum where we can all get together, share the latest advances in our research and discuss new methodologies that are coming out of our laboratories that could be useful for other people.
What is exciting about this meeting is that often people are presenting work that has not yet been published and may not be published for six months, a year or even longer, so you have the opportunity to see work long before the rest of the world does.
I think the less obvious thing with a meeting like this one is that you also have an opportunity to interact a little bit more informally with other participants, so you can get into detailed conversations about work that you are doing that may or may not make it into a formal presentation. I think the personal contact is a very important part of this meeting.
What direction do you see, or would like to see, AFM going in the next five years? (What do you see as the next big thing for AFM?)
As I mentioned, one major contribution that Bruker has made is trying to integrate atomic force microscopy technology with other types of technologies that are valued by life scientists.
I would love to see even more progress in that direction from Bruker and other AFM manufacturers. I would like to see them figure out how to integrate AFM measurements with biochemical or genetic measurements that are pushing life sciences forward in other areas and make AFM measurements more high-throughput for the purpose of incorporating them into the drug screening and discovery pipeline.
I think, historically, one limitation with atomic force microscopy is that, although it is very high resolution, sometimes setting up an experiment is not trivial. Data acquisition can take a very long time. I think anything that can be done to try to bring it up to speed with other technologies in that regard would be a major advance. I would love to see the field go in that direction.
Where can readers find more information?
About Prof. Sanjay Kumar 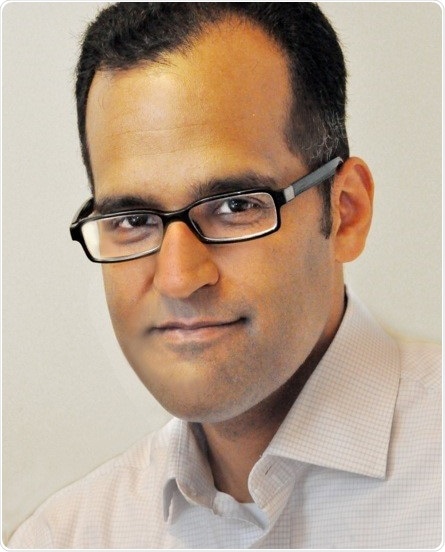
Sanjay Kumar, M.D., Ph.D., is Professor and Associate Chair of BIoengineering at UC Berkeley, where he has been a faculty member since 2005. He is also Professor of Chemical and Biomolecular Engineering at UC Berkeley and a Faculty Scientist in the Biological Systems & Engineering Division of Lawrence Berkeley National Laboratory.
Dr. Kumar earned his B.S. in Chemical Engineering at the University of Minnesota (1996) and his M.D. and Ph.D. in Molecular Biophysics from Johns Hopkins University (2003). Prior to joining the UC Berkeley faculty, he completed postdoctoral training at Boston Children’s Hospital and Harvard Medical School.
He and his research group have been fortunate to receive a number of honors, including the Presidential Early Career Award for Scientists and Engineers (PECASE), The NIH Director's New Innovator Award, The Arnold and Mabel Beckman Young Investigator Award, the NSF CAREER Award, and the Stem Cells Young Investigator Award.
Dr. Kumar has also received awards by student vote for Excellence in Graduate Advising (UCSF/UC Berkeley Joint Graduate Group in Bioengineering) and Outstanding Teaching (Bioengineering Honor Society) and has served as a Presidential Chair Teaching Fellow. Dr. Kumar is an elected Fellow of AIMBE and BMES.