Sponsored Content by PittconMay 6 2020
In this interview, Dr. Nancy Allbritton from The Allbritton Lab talks to News-Medical and Life Sciences about the revolutionary technology and techniques for the application of new technologies for oncology and stem cell research.
Dr. Nancy Allbritton provides insight into the organ-on-a-chip and the ability it has to monitor and control the environment at the cellular and tissue level is one of the most promising applications for microengineered systems. Dr Allbritton will be presenting her research and findings at Pittcon 2020 in Chicago.
What are microphysiological systems (organs-on-chips) and why are they becoming increasingly important in biology and medicine?
Microphysical systems seek to replicate the smallest functioning unit of an organ. For intestine-on-a-chip, it might be large or small intestinal crypts. For a heart, it may be a section of contractile muscle. For the liver, it may be a liver lobule or group of lobules. It's a group of interconnected cells so that they are not just behaving as a single cell or just a small cluster, but they began to show higher-order behavior and functioning.
I think one of the reasons microphysical systems are becoming increasingly important is that we've had a lot of breakthroughs in stem cell technology. The ability to grow human stem cells from different organs is now present, as well as the ability to create a tissue of differentiated cell types from primary stem cells or organ-specific cells from induced pluripotent stem cells.
Right now, it is the perfect time for this technology, because we have all the enabling microfabrication methods and stem cell biology innovations coming together. Another reason organs-on-chips are becoming important is the ability to grow human tissue. It's very hard to do experiments on the human population and get a good representation of the population, either because people aren't volunteering or because there just aren't enough people in that particular group of individuals. With the chip, you can begin to sample population-wide tissue variation. Organs on chips can also be a better way to test drugs. Rather than testing cells in a dish, which are usually tumor cells and very abnormal in their growth and other characteristics, organs-on-chips constructed from normal human cells can be used to get a more accurate representation of how drugs will affect humans. Additionally, they may outperform murine experiments in many ways since humans are not simply 70 kg mice.
We have been able to cure mice of all sorts of diseases, but much of this work hasn’t translated to humans. So, while that was a good screening technology, a lot of drugs got through that were quite toxic to humans, and then a lot of drugs that may have worked well in humans, but were toxic to mice got blocked.
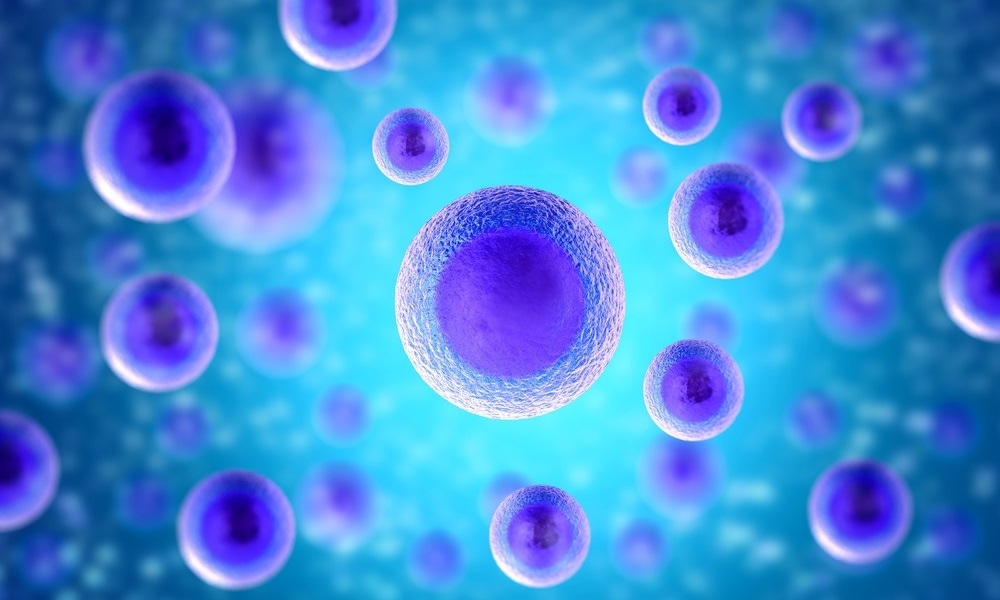
Image Credit:Shutterstock/royaltystockphoto.com
Microphysiologic devices should maximize the number of good drugs that could work well in humans. You can hopefully also get higher value information and create a much better drug pipeline. I think doing high-throughput screening on organ subunits on microfabricated devices can complement drug screening in humans. The idea is that you may be able to cut costs in several ways;
- reducing the number of animal studies
- removing ineffective drugs from the pipeline earlier (bad drugs fail earlier in the pipeline)
- minimizing the extent to which human studies are necessary
I believe you can also begin to develop human disease models. You can create mouse models of human disease, but they almost never completely recapitulate human disease. Even when it is a simple genetic mutation, the mice can often be asymptomatic or present with different symptoms and outcomes to that mutation. With the organ-on-a-chip, you can have functioning human tissue that mimics many of the symptoms of human diseases. Even more exciting, one can begin to put, for example, an intestine-on-chip coupled with the human gut microbiome or the gut flora and begin to understand how the tissue and microbiota interact.
This is important because our gut flora is very different from that of a mouse and other model organisms. We now know that intestinal microbes have a tremendous physiologic impact throughout our body including metabolism and mentation, our feeding behavior, and these are aspects of human behavior or physiology that can't be recapitulated in an animal model or cells in a dish.
Eventually, we'll be able to string human organs together as human organ-on-chip systems. For example, food is added to the gut model and absorbed, the nutrients travel to the liver, the liver metabolizes the absorbed compounds sending them out to the body as a whole including the brain and heart. The organs must be tied together to see the full effect in which the behavior or functioning of one organ impacts other connected organs. That will never replace a fully functioning human, but I think the expectation is that it may end up being a lot more accurate and, in some ways, cheaper than mice or other model mammalian systems.
The organ-on-a-chip systems will help us understand basic biology and the basic physiology of the human.
Why are new engineering methods needed for microphysiological systems?
Microphysiologic devices depend on many fields of chemistry for their advancement including synthetic organic, polymer and analytical chemistry. We need more synthetic matrices to support cells and tissues including smart polymers and scaffolding materials that will support, direct and shape these organ systems. Right now, people often use matrices derived from native biologic materials, like collagen or matrigel, which are expensive and not fully defined. Polymer chemists and synthetic chemists are working hard to develop novel materials and matrices while analytical chemists, as well as engineers, are developing materials and methods for microdevice fabrication, sensor enhancements, and other device-related innovations.
Microphysiologic systems will clearly need an array of embedded and external sensors to monitor their health and well-being as well as pathophysiologic attributes. They will need robust, reliable (and often miniaturized) embedded sensors for oxygen consumption, glucose concentration, CO2 production, pH, and other chemical and physical attributes. Importantly the sensors should not perturb the system. These sensors will likely require external instrumentation or detection methods to monitor the embedded sensors, for example, RFID or optical readouts.
For engineering and chemistry, these are going to be huge areas where many can contribute to moving the field forward. For engineering, specifically, developing ways to make integrated systems that are efficient, low cost, manufacturable, shippable, self-contained, and can talk to each other will be important. This is an area where engineering and chemistry must work together in a team approach to move the field forward. It's going to take both disciplines to really advance the field.
What is microfabricated technology and how can it be used to overcome these issues?
Microfabricated technology refers to devices developed with micron-sized features. Cells have diameters on the order of 10 microns and many organ subunits span hundreds of microns so that micron-sized architectural features are required to recapitulate key features. For example, large intestinal crypts (the key physiologic subunit of the large intestine) is approximately 400 microns in length and 100 microns wide with the stem cell niche spanning tens of microns. Thus, microfabrication methods are perfect for rebuilding many of the key architectural features of the large intestine.
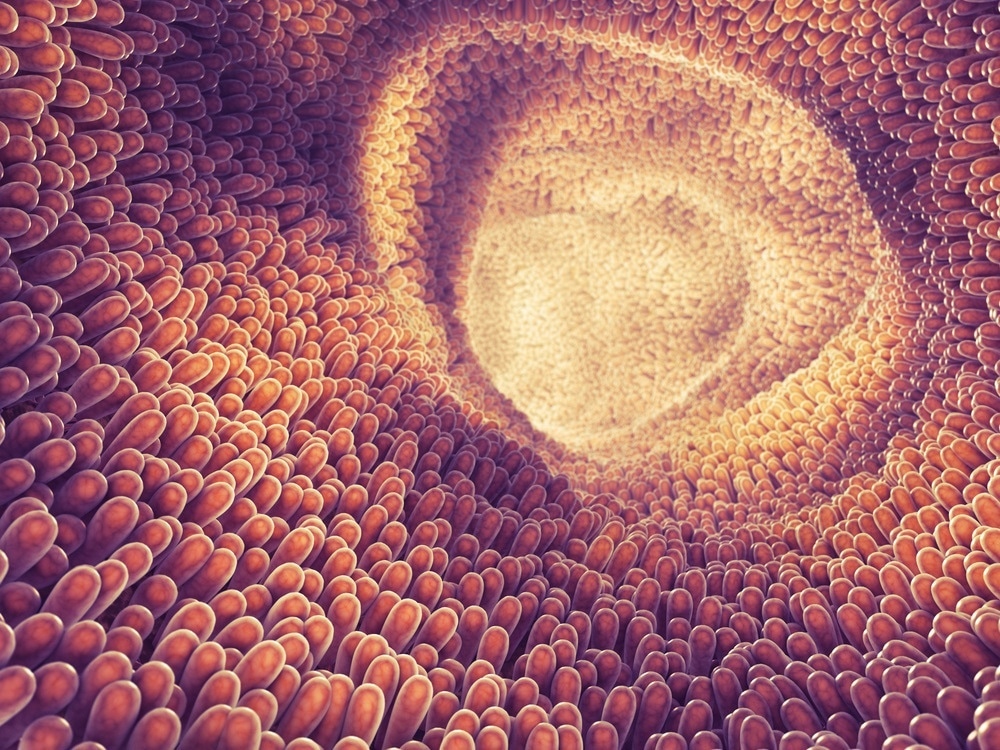
When it comes to rebuilding architectural features such as the lare intestine, microfabrication methods are perfect for this. Image Credit:Shutterstock/nobeastsofierece
How were the microfabricated platforms used in your lab developed?
We do intestine-on-a-chip, which is mostly the large intestine and have several platforms of varying complexity. The simplest is the human intestinal epithelial monolayers that have a stem and/or differentiated cells. These can be used to assess how the intestine transports and metabolizes drugs and nutrients or how the stem cells differentiate into mucus-producing, hormone-producing, or absorptive cell types. These systems are designed to be higher throughput model systems that are simple but do not possess the greatest possible information content. However, they are robust and reliable. We also have complex 3D tissues that replicate a wide range of physiologic behaviors as well as the architectural features of the human intestine. Importantly many of these systems will support the wide array of chemical and gas gradients found in the human intestine. They can also host the human microbiome so that a better understanding of the complex interplay between the human cells and microbes can be developed in both health and disease.
As with all of our model systems, we usually advise users of our systems to employ the simplest platform possible and then add in complexity as needed for the task at hand.
How have you used the platforms to create structures that resemble tissues in vivo?
We have more complex platforms that are designed to replicate a lot of the different features of the human intestine, such as the architecture, cell migration behaviors, and stem cell fate decisions. These are shaped three-dimensional systems that exist as arrays of crypts (or microwells) covered with a monolayer of intestinal epithelial cells. The crypt array has a basal surface for a nutrient diffusion, and a lumen, like the inside of the intestine.
You can imagine the cells on the inside of the intestine, facing the waste or food, are very different from the cells that are at the base of the intestinal crypts. In these systems, the stem cells are found at the crypt or microwell base while the differentiated, mature cells such as absorptive cells are found facing the luminal surface, i.e. waste side. These systems are chemically and architecturally much more faithful duplicates of the human intestine than the simpler systems, but that also means they're a little harder to create, build, and maintain. With some of these three-dimensional systems, we create chemical gradients across the tissues, i.e. the long axis of the intestinal crypt, just as stem cell factors and food/bacterial metabolites exists in a gradient across your intestinal crypts. This microdevice permits very sophisticated micro-environmental control of these complex tissues.
Living tissues and organs are highly dynamic. Are you able to replicate the extracellular matrix and extracellular signals that would normally exist within an organ?
We can do a lot of it, but we can't do it all. For example, with the chemical gradients, the stem cells that sit at the base of these microwells are exposed to very high growth factors, but about 400 microns away, so a few hair diameters, the cells don't see much of these factors. This chemical gradient mimics the signaling microenvironment of the intestine very closely. There are also all sorts of bacterial metabolites and products that are at very high concentration for the cells at the luminal surface, but low where the stem cells are down at the base of the microwells or crypts. We can also replicate these food and bacterial metabolite gradients by using purified compounds or actual bacteria on the luminal side of the intestine on a chip.
This 3D system begins to duplicate the human physiologic signals that control the cells’ behavior, but there are some things we cannot do yet. We don't have a blood supply, for example, or blood vessels going into our chip. That’s one of the next steps, but there is a lot of work to do to build it, yet still have a robust and reliable intestine-on-chip system. Our tissue is growing in complexity, but we still only have 1 to 2 tissues on the devices and a small subset of the bacteria found in the human intestine. One could imagine increasingly putting more and more tissue types. The intestine has epithelial cells, fibroblasts, muscle cells, neural cells, immune cells, all sorts of other cell types, and adding in all of those cell types would create a more normal physiologic organ. I think those are all goals for the future on which we will be moving forward.
How could the intestinal monolayers you have developed in your laboratory be used for drug development?
Our intestinal-on-chip technologies can be used to understand drug transport and metabolism by humans (as opposed to mice or tissue-cultured tumor cells). Additionally, the microbes in the human intestine or the human intestinal epithelium can convert drugs into their active form or into a toxic metabolite. Along that line, one could begin to do rapid screens as to how drugs are modified, metabolized and transported across the intestinal epithelium. Importantly many drugs cause intestinal dysfunction or side effects and screens can be performed for the impact of the drugs on the intestinal cells themselves and the impact on intestinal barrier function, e.g. leakiness.
As an example, it's now also clear that in cancer chemotherapy, the gut and the bacteria within the gut play a huge role in how well the chemotherapy is working. How this works is not well understood, so I think there's going to be a big push to use these systems to understand how we can make anti-cancer drugs that are more effective and less toxic on the gut and other biological systems. We are only beginning to scratch the surface.
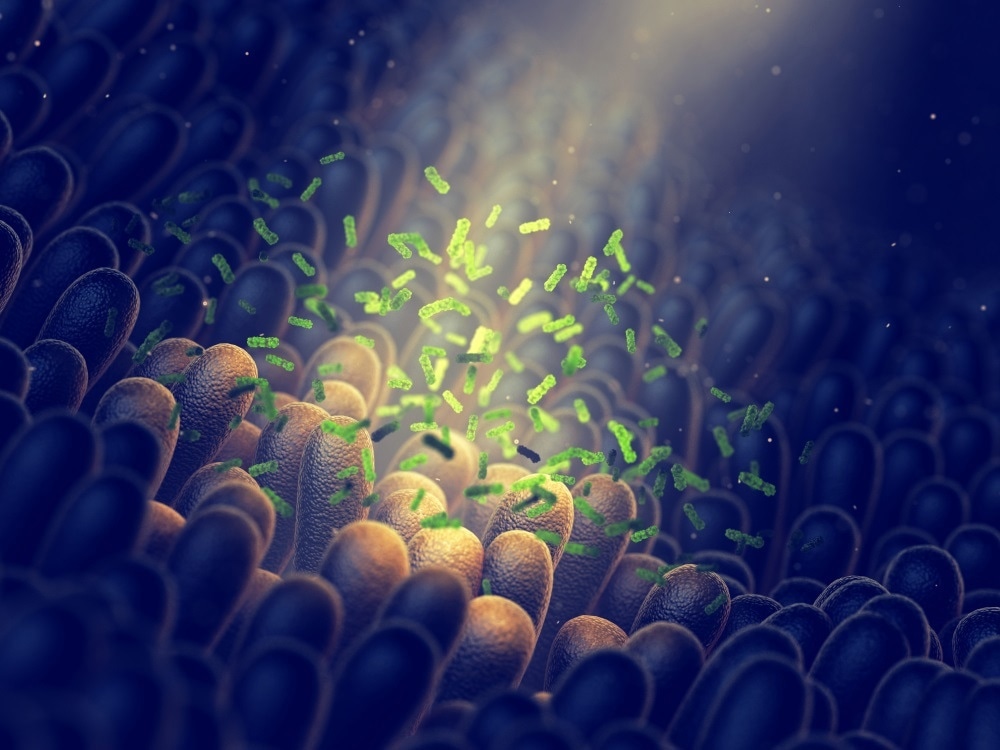
Image Credit:Shutterstock/ nobeastsofierce
Do you think that microphysiological systems could one day replace animal models? What challenges will we need to overcome before this is possible?
I don’t think microphysiological systems can ever totally replace animal models. It's the law in the US to test drugs on animals. We are also a long way off from having a full human-on-a-chip with all of the different organ systems in place and interconnected, whereas a mouse or other animal system is already there. I think it's more likely that the chips will reduce the number of animals used so that you can get higher value information, thereby complementing rather than replacing animal models. The chips will also enable insights as to how humans might ultimately respond differently from animal model systems.
On-going work in the organ-on-chip area is to demonstrate that these devices actually mimic and replicate human responses (and many have already been shown to mimic human physiology when animal models failed). Overall the future for organ-on-chip technologies appears quite bright and will undoubtedly grow in future importance and impact.
How far are we away from building a microphysiological system that could be used to study complex diseases, such as cardiovascular disorders?
There are already devices that demonstrate many of the complex disease phenotypes. For example, blood vessels on-chip have replicated blood vessel diseases such as atherosclerosis and tumor metastases. There are some amazing heart-on-chip devices with functioning cardiac tissue subunits that accurately recapitulate the impact of cardiovascular drugs, so I think we are making good progress. I think that we will see a growing number of impressive and highly predictive disease models as time goes by.
Finally, what does the future hold for you and your research?
Near term, our big goal is to really make an intestine-on-a-chip that fully replicates the human small and large intestines. We're working hard at putting a human microbiome or normal intestinal bacteria on our intestine-on-chip. In addition to chemical gradients, gas gradients such as oxygen exist across the intestinal crypts which we're working hard at replicating. We really want to add in more tissue types, such as the immune system, fibroblasts, and nervous tissue. We've got quite a bit of work to do to make a fully functioning replica so we are also starting to team up with other people, particularly liver-on-a-chip people so that our intestine-on-a-chip can absorb food and then send it to their liver-on-chip to create a fully functioning liver-intestinal model to recapitulate food digestion, metabolism, and detoxification.
A big part of our work is to make the systems robust and reliable as well as easy to use for biologists and clinical investigators. That may sound trivial, but it's not. A lot of great devices remain isolated in the inventors' lab due to their high degree of complexity. When biologists try to use these complex devices, there are just too many failure points. Even to make one of these systems shippable will be a challenge, because how do you give it to FedEx, yet arrive in good condition across the world? There are a lot of challenges with scalability, manufacturing, robustness, and reliability that my lab, in particular, is interested in tackling to make sure that these organ-on-chips get out into the real world and fulfill their potential.
Where can our readers go to find out more?
To find out more please visit https://its.unc.edu/
About Nancy Allbritton
Dr. Allbritton is a Professor in the Department of Bioengineering and the Frank & Julie Jungers Dean of Engineering at the University of Washington in Seattle. She has been the scientific founder of four companies and enjoys international travel.
About Pittcon
Pittcon® is a registered trademark of The Pittsburgh Conference on Analytical Chemistry and Applied Spectroscopy, a Pennsylvania non-profit organization. Co-sponsored by the Spectroscopy Society of Pittsburgh and the Society for Analytical Chemists of Pittsburgh, Pittcon is the premier annual conference and exposition on laboratory science.
Proceeds from Pittcon fund science education and outreach at all levels, kindergarten through adult. Pittcon donates more than a million dollars a year to provide financial and administrative support for various science outreach activities including science equipment grants, research grants, scholarships and internships for students, awards to teachers and professors, and grants to public science centers, libraries and museums.
Visit pittcon.org for more information.