The severe acute respiratory syndrome coronavirus 2 (SARS-CoV-2), the causal agent of the coronavirus disease 2019 (COVID-19) pandemic, has infected over 448 million and caused over six million deaths worldwide. Each new SARS-CoV-2 infection provides the opportunity for multiple genomic mutations to arise during the replication process, which results in the production of new variants with new characteristic features, such as increased transmissibility and virulence.
Owing to mutations in the SARS-CoV-2 genome, several major waves of infection have occurred. These have included COVID-19 waves due to the emergence of the SARS-CoV-2 Delta variant (B.1.1.617.2) and, more recently, by the Omicron variant (B.1.1.529).
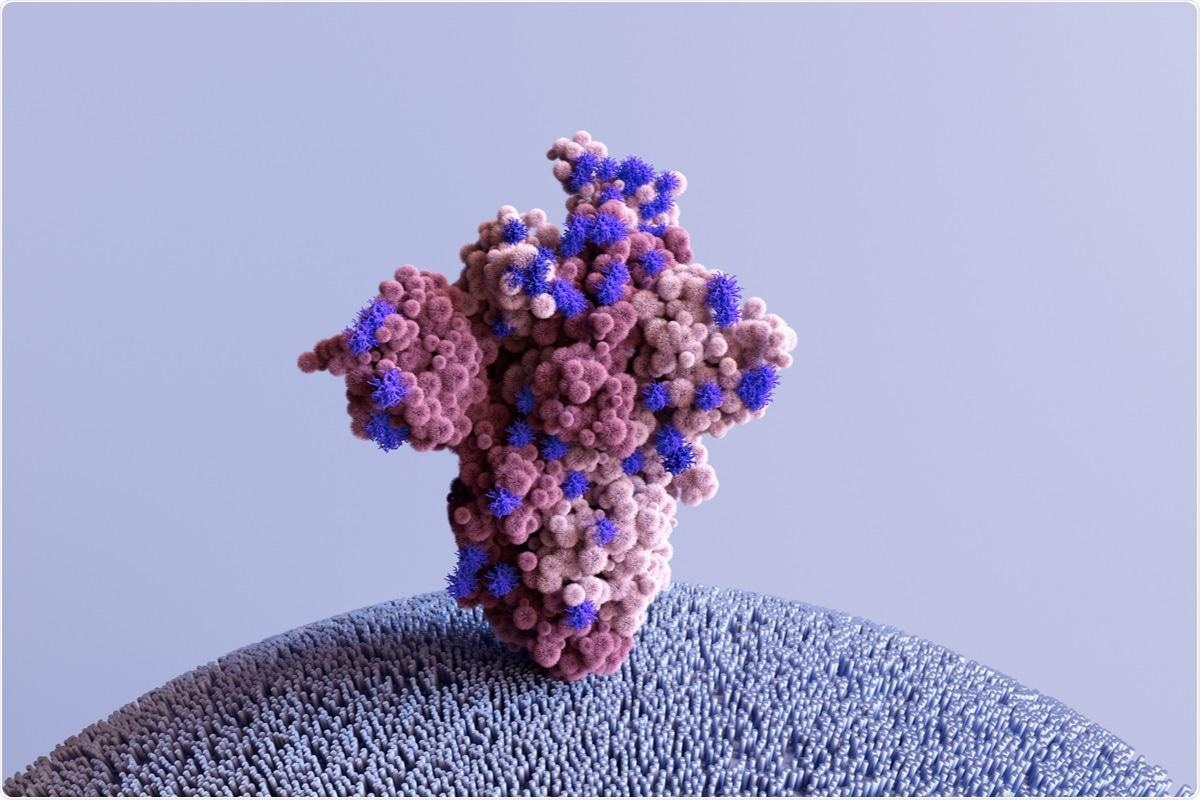
Study: The Future of the COVID-19 Pandemic: How Good (or Bad) Can the SARS-CoV2 Spike Protein Get? Image Credit: Design_Cells / Shutterstock.com
The SARS-CoV-2 spike (S) protein plays an important role in viral infection; therefore, it is imperative to study the structural and functional limits of the S protein, to prepare for future SARS-CoV-2 variants. A new Cells review paper discusses the factors that affect the function of the SARS-CoV-2 S protein, while also providing insights into the theoretical limits of this viral protein.
S protein structure and function
The SARS-CoV-2 S protein is around 180 kDa in size and is expressed as a trimer on the surface of SARS-CoV-2. SARS-CoV-2 typically consists of approximately twenty-five S trimers in each virion.
The S protein binds with the human transmembrane angiotensin-converting enzyme 2 (ACE2) receptor to establish the viral infection. As ACE2 is also highly expressed in many tissues including oral epithelia, enterocytes (small intestine), and the vascular endothelium, it enhances the possibility of SARS-CoV-2 infection through various routes, aside from its primary entry through the respiratory route.
The S protein from the original SARS-CoV-2 strain reported in 2019 in Wuhan, China consisted of 1,273 amino acid residues arranged into two subunits known as S1 and S2. The S protein also houses the receptor-binding domain (RBD), which contains a receptor-binding motif (RBM) in the S1 subunit that primarily interacts with the ACE2.
SARS-CoV2 Infection. (1) Spike proteins (red) on the SARS-CoV2 surface bind to ACE2 (blue) on the surface of target host cells. (2) Spike-ACE2 interactions position Spike for proteolytic processing on the cell surface by TMPRSS2 (orange) (or by cathepsin in endosomes after internalization). Cleavage of Spike releases a structural constraint that allows Spike to convert to its “fusion” structure that penetrates the host cell membrane. (3) “Fusion” Spike facilitates fusion between the viral and host membranes, allowing entry of viral RNA. (4) Viral RNA is translated and replicated within the host cell. (5) New virus particles are packaged in the host cell where furin cleaves Spike so as to prime it for later conversion to the “fusion” structure. (6) New SARS-CoV2 particles are released (or transferred to adjacent fused cells) to propagate further host cell infection.
The RBD has a flexible structure that can dynamically alter between an open (up) and closed (down) conformation. Notably, the S protein can infect the host well only when RBD is in the open conformation.
The S protein binds to ACE2, which is present on the host cell surface. This binding event is followed by cleavage of the S protein by the transmembrane protease serine 2 (TMPRSS2).
TMPRSS2 facilitates fusion of the viral and host cell membranes, which results in the entry of viral ribonucleic acid (RNA) into the host cell, where it is further translated and replicated to produce new viral particles. Variations in ACE2 expression based on gender, age, and disease have been observed, which explains the clinical differences in SARS-CoV-2 infection.
Except for the RBD, the S protein is heavily glycosylated, which provides wide shielding from antibody recognition. The glycosylation of the S protein affects the transition between the open and closed state of the RBD.
At an open conformation, glycans at N165 and N234 stabilize RBD, whereas the glycan at N343 pushes the RBD up into the open state. Mutations at any of these three residues have been shown to reduce the binding of the S protein to ACE2; however, the loss of the glycan at N343 reduced binding by more than 50%.
Side and top views of Spike trimer with site-specific glycosylation shown (moss surface) from molecular dynamics simulations [23]. The glycans are shown in ball-and-stick representation in green, dark yellow, orange and pink. The protein surface is colored according to antibody accessibility from black (least) to red (most accessible). One RBD is shown in the open conformation (circled in blue). Images are from [23] and are used under Creative Commons Attribution 4.0 International License: https://creativecommons.org/licenses/by/4.0/, accessed on 1 March 2022.
Mutations in the S protein
Previous studies have revealed that the SARS-CoV-2 Alpha, Beta, and Gama variants contain the N501Y mutation within the RBM. This mutation has enhanced the binding affinity of these variants to ACE2 by three- to seven-fold.
The Delta and Omicron variants, which are two of the most contagious variants of SARS-CoV-2 to date, contain additional mutations. For instance, the Delta variant contains two the L452R and T478K mutations in the RBM region, while the S protein of the Omicron variant contains thirty-point mutations, three deletions, and one insertion. More specifically, the Omicron variant contains ten tightly clustered mutations in the RBM that include N440K, G446S, S477N, T478K, E484A, Q493K, G496S, Q498R, N501Y, and Y505H.
In addition to ACE2, scientists have determined the presence of secondary binding sites for SARS-CoV-2 such as heparan sulfate proteoglycans (HSPGs). The presence of additional binding sites significantly enhances the ability of host cells to bind with SARS-CoV-2 and, thereby, increases the risk of viral infection.
Evolution of SARS-CoV-2 S protein
Scientists have used enzymology to estimate the maximum theoretical catalyzing rates of chemical reactions. Moreover, upon analysis of the binding affinity of the Omicron S protein to ACE2, researchers have found reduced affinity as compared to the Delta variant. However, in the case of the Omicron variant, a tight complex with ACE2 is mediated by HSPG co-receptors.
As a result of various unknown factors, the evolution of S proteins could not be predicted. More models are required to accurately measure the number of ACE2 and TMPRSS2 molecules, as well as heparan sulfate binding sites per cell. New models are required to identify processes that new SARS-CoV-2 variants might exploit to increase their virulence and transmissibility.
Journal reference:
- Nugent, M. A. (2022) The Future of the COVID-19 Pandemic: How Good (or Bad) Can the SARS-CoV2 Spike Protein Get? Cells 11(5). doi:10.3390/cells11050855.