Both the severe acute respiratory syndrome coronavirus 1 (SARS-CoV-1) and SARS-CoV-2 have led to the SARS epidemic and coronavirus disease 2019 (COVID-19) pandemic, respectively, within the last two decades.
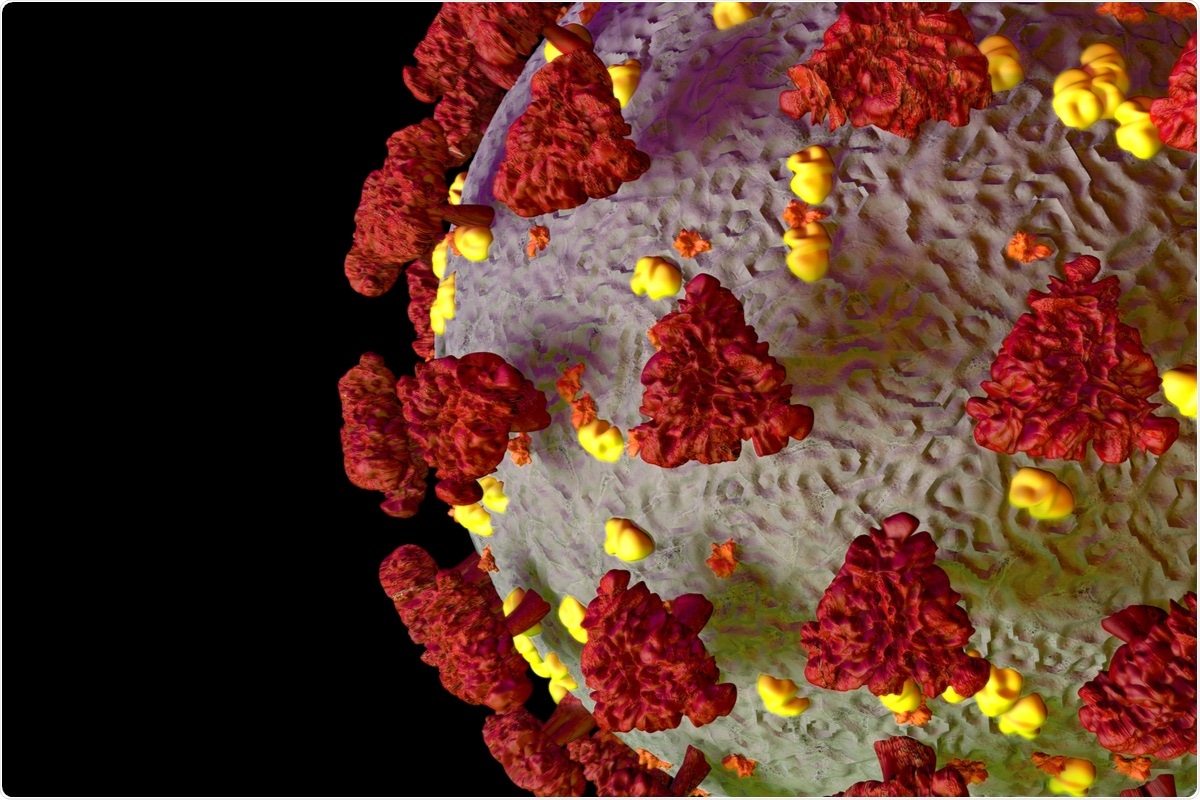
Study: Prefusion Spike Protein Conformational Changes Are Slower in SARS-CoV-2 than in SARS-CoV-1. Image Credit: 168 STUDIO / Shutterstock.com
Background
Although several studies have shown that the transmissibility of SARS-CoV-2 is higher than SARS-CoV-1, both of these coronaviruses share highly similar structural and functional features.
For example, the spike protein plays an important role in the infection process for both SARS-CoV-2 and SARS-CoV-1. Moreover, the SARS-CoV-1 and SARS-CoV-2 spike proteins have a high sequence identity of approximately 79%.
The receptor-binding domain (RBD) of both spike proteins interacts with the human angiotensin-converting enzyme 2 (ACE2) receptor. Several studies have indicated that many regions of the SARS-CoV-2 spike protein are susceptible to mutations, with RBD being the most vulnerable.
Therefore, therapeutics that target the RBD-ACE2 interaction might become effective over time due to emerging variants with mutations in this region. Increasing the efficacy of therapeutics and vaccines by diversification of the hot spots of targeted protein is thus required.
Recent studies using cryogenic electron microscopy (cryo-EM) and computational approaches have highlighted the differential receptor binding behaviors of the SARS-CoV-1 and SARS-CoV-2 spike proteins. To this end, the RBD appears to undergo a conformational transition from an inactive “down” position to an active “up” position to access the ACE2 receptors that are located on the surface of host cells.
Several experimental studies on the binding affinity of the spike protein RBD for the ACE2-peptidase domain (PD) have produced varied results. Thus, there remains an urgent need to improve the understanding of the RBD-ACE2 interaction.
About the study
In a new Journal of Biological Chemistry study, researchers aimed to determine the differential dynamic behavior of SARS-CoV-1 and SARS-CoV-2 spike proteins by using an extensive set of equilibrium and nonequilibrium microsecond-level all-atom molecular dynamics (MD) simulations. The study also involved extensive steered MD (SMD) simulations, along with nonequilibrium work calculations for semi-quantitative comparison between the two spike proteins.
The current study included MD simulations that were based on cryo-EM structures of the SARS-CoV-2 and SARS-CoV-1 spike proteins in their active and inactive states. A 5-μs-long unbiased all-atom MD simulation was carried out in explicit water for both inactive and active SARS-CoV-1 and SARS-CoV-2 spike proteins. The active SARS-CoV-1 and SARS-CoV-2 simulations were repeated for another 5 μs each.
A total of 80 independent nonequilibrium SMD simulations were also performed to compare the spike proteins of both SARS-CoV-2 and SARS-CoV-1.
Study findings
Within the time of the unbiased equilibrium simulations, no conformational change of the inactive forms of the SARS-CoV-1 and SARS-CoV-2 spike proteins was observed. The RBDs were also found to remain in the “down” position.
A spontaneous large-scale conformational change was observed in the active SARS-CoV-1 spike protein simulation, where the RBD transitions from an active “up” position to a pseudo-inactive “down” conformation. However, no such conformational transitions have been observed in the SARS-CoV-2 spike protein.
Root mean square deviation (RMSD) and root mean square fluctuation (RMSF) analyses have shown that the stability of active SARS-CoV-2 is higher as compared to the SARS-CoV-1 spike protein. The center-of-mass distance between the receptor-binding motif (RBM) of protomer A and the S2 trimer of the spike protein was found to be stable for both inactive states, while it decreased for the SARS-CoV-1 active state. Additionally, the angle between the RBM of protomer A and the S2 trimer was found to change for the SARS-CoV-1 active state but not for the SARS-CoV-2 active state.
The distance between RBD and N-terminal domain (NTD) of protomer A decreased for the SARS-CoV-1 active state, which suggests that the pseudo-inactive conformation adopted by the active SARS-CoV-1 spike protein is unique. No such observations were made for the active SARS-CoV-2 spike protein or inactive spike proteins. The water molecule state for the pseudo-inactive state was also found to be lower than the SARS-CoV-2 active state and comparable to SARS-CoV-1/2 inactive states.
The driving force behind the conformational transition observed in the initially active SARS-CoV-1 spike protein simulation was a set of salt-bridge interactions that were unique to CoV-1. Unique salt bridge interactions, which have also been observed in SARS-CoV-2, appear to contribute to the relative stability of the active SARS-CoV-2 spike protein.
Additionally, conformational changes that were associated with the activation or inactivation of spike protein proceeded more slowly in SARS-CoV-2 as compared to SARS-CoV-1. The SMD simulations also showed that it was difficult for the SARS-CoV-2 spike protein to undergo a large-scale conformational transition between active and inactive states as compared to SARS-CoV-1.
Conclusions
The current study demonstrates the conformational dynamics of the SARS-CoV-1 and SARS-CoV-2 spike proteins. Along with differences in dynamic behaviors, factors such as spike protein glycosylation and the behavior of other viral proteins may also contribute to the difference in transmissibility and infectivity between these two viruses.
Therefore, additional computational and experimental studies are required to accurately determine the differential infectivity and transmissibility of SARS-CoV-1 and SARS-CoV-2.