For many decades, access to nuclear magnetic resonance (NMR) spectroscopy has been an essential requirement for analytical chemistry laboratories.

Image Credit: Oxford Instruments NMR
This technique’s ability to highlight molecular structure and track reaction dynamics has garnered widespread recognition throughout academia and industry as a robust, nondestructive, noninvasive method taught in virtually all undergraduate chemistry curricula.
Widespread access to NMR has been challenging. The technology has historically required a great deal of space, expert users, and specialized facilities, limiting its usability in synthetic chemistry and industrial labs.

Image Credit: Oxford Instruments NMR
Current advances in benchtop NMR instrumentation have been key to bringing this powerful technique out of the centrally managed facility or basement and into the heart of virtually any laboratory.
Benchtop NMR instruments offer a more compact, cryogen-free version of conventional high-field instruments. They can even collect data in a fume hood.
Oxford Instruments’ X-Pulse adds powerful broadband capability to benchtop NMR, allowing operators to employ a single benchtop instrument, rapidly tuning this to accommodate most NMR active chemical nuclei.
The instrument can be shared across an array of applications, futureproofing the laboratory’s research and development capabilities and considerably expanding the potential to address challenges across academia, education, and industry.
The benefits of mobile, small-footprint benchtop NMR for the industry are clear, including reduced preparation times, fewer specialist staff, automation capabilities, and fewer demands on available space.
Benchtop NMR systems offer notable cost savings and time efficiencies when compared to their high-field NMR counterparts, which typically require substantial initial capital investment and dedicated rooms to operate within.
Benchtop NMR can be situated alongside existing instruments or transported on a trolley between labs where required. Because it is cryogen-free, it is also much less expensive to run than conventional high-field instruments.
Oxford Instruments' analyses highlighted that it is possible to save more than $9,000 USD per year solely by eliminating the need for cryogens.
Businesses considering investing in high-field NMR should consider several factors. For example:
- Will it be possible to establish and maintain a reliable supply of liquid helium and liquid nitrogen?
- How will these materials be safely stored once on site?
- Will a sufficiently qualified member of staff be on hand to handle these cryogens in a safe fashion?
The cost of cryogens continues to rise, and logistical challenges remain regarding buying and installing a high-field instrument.
Research and development
Early applications were primarily focused on rudimentary one-dimensional 1H NMR spectra, but contemporary spectrometers offer a number of additional capabilities in addition to their capacity for structural elucidation.
For example, modern spectrometers can measure self-diffusion coefficients to extract physical information about a sample. This information includes molecular size and viscosity, as well as ionic conductivity and transference, for example, in lithium-ion battery electrolytes.


Figure 1. A sample of the various X-nuclei that it’s possible to detect on X-Pulse. Image Credit: Oxford Instruments NMR
When a benchtop NMR spectrometer is fitted with pulsed field gradient (PFG) hardware, it can leverage techniques such as the PFG spin echo (PFGSE).
This experiment is ideally suited to determining the diffusion coefficients of sample components, achieving this by measuring changes in the NMR signal as a function of the PFG strength. The addition of variable temperature capabilities enables the study of samples’ thermal behavior under a range of anticipated working conditions.
It is also possible to apply pulsed field gradients to the sample in varying intensities. As gradient strength increases, the signal will be attenuated as a result of the changing phase difference between molecules following the first and second gradient pulses.
The Stejskal-Tanner equation can be used to relate this attenuation to the diffusion constant.1
This method is especially useful in battery research and development. Measuring various lithium salts’ cationic transference, self-diffusion, and ionic conductivity in various electrolytes can help quantify performance and enhance the battery’s design.
In the example presented here, lithium hexafluorophosphate—Li[PF6]—was analyzed in three different electrolyte solvents: dimethyl carbonate (DMC), a 50:50 mixture of ethylene carbonate (EC) and DMC, and propylene carbonate (PC).

Figure 2. Stejskal-Tanner plots of integrals obtained from PFGSE data of LiPF6 in DMC, 50:50 EC:DMC, and PC. 1H data is shown in dark orange and light orange 7Li data is shown in blue, 19F in grey, and 31P in green. All data were acquired on a single X-Pulse broadband benchtop NMR spectrometer. Image Credit: Oxford Instruments NMR
Figure 2 shows the resulting PFGSE spectra from this analysis, highlighting the differences in diffusion behavior between the components of a single electrolyte solution. These spectra also illustrate the range of differences between the same species in different solvent conditions. It is important to note that 31P is part of the same [PF6]− ion as 19F, meaning that the two should diffuse at an identical rate.
These diffusion behaviors were found to be identical within the method’s precision but, as anticipated, the smaller Li+ ion’s diffusion behavior was notably different from the behavior of the larger [PF6]− ions.
Table 1 displays distinctly different quantitative results for the same Li+ and [PF6]− ions in the three solvent systems. This highlights the importance of solvent choice when designing batteries.
In the example presented here, conductivity was found to differ by approximately a factor of three between electrolytes using PC and DMC solvents, while cation transference was found to alter much less.
The differences in diffusion behavior for DMC as a pure solvent versus that of the 50:50 mixture with EC highlight the impact of environmental factors on solvent molecules.
Leveraging benchtop NMR’s portability allows these electrolyte characterizations to take place in the lab, enhancing and accelerating development capabilities from the beginning of the process.
Table 1. Parameters measured by NMR for three electrolyte systems: LiPF6 in DMC, in 50:50 EC:DMC, and in PC. Source: Oxford Instruments NMR
Sample |
D+, cation |
D-, cation |
D, solvent |
Conductivity |
Transference (t+) |
|
X10-10m2s-1 @39.5c |
X10-3Sm-1 |
|
DMC |
5.16 |
5.68 (19F)
5.81(31P) |
12.7 |
3.91 |
0.48 |
EC:DMC |
2.74 |
4.45 |
6.42 (EC)
7.38 (DMC) |
2.54 |
0.38 |
PC |
1.24 |
2.30 |
2.56 |
1.28 |
0.35 |
Quality assurance/quality control
As well as offering a robust tool for research and development, benchtop NMR has powerful quality assurance/quality control (QA/QC) potential. A key example of these capabilities can be found in the food industry, where NMR is widely used in the identification of impurities or adulterants.
Coffee is one of the most widely traded commodities in the world, and this industry significantly benefits from NMR technology. The coffee trade is primarily comprised of two varieties: arabica (C. arabica) and robusta (C. canephora).
Arabica accounts for 60-70% of the global market and is widely regarded as of higher quality, selling on world commodity markets for approximately twice the price of robusta.
This difference opens up the potential for economic fraud, with dishonest traders labeling a mix of robusta and arabica as “100% Arabica” to sell this at a higher price. It is, therefore, necessary to employ appropriate analytical methods to detect the presence of robusta coffee in products labeled as arabica.
DIN 10779 is the official method for investigating the presence of robusta in arabica, but this complex HPLC method takes more than seven hours to complete and includes the use of acetonitrile, a known carcinogen.
Defernez et al. have highlighted that low-field (benchtop) NMR can be used in place of the methods outlined in DIN 10779, discovering that it was possible to detect 16-O-methylcafestol (16-OMC) to provide a more rapid, sustainable means of detecting adulteration of arabica by robusta.2
This method was used in a surveillance study of retail-purchased “100% Arabica,” revealing that 6 of the 60 samples surveyed showed signals corresponding to adulteration at levels of 3-30% w/w2.
16-OMC exhibits a number of clear peaks in the NMR spectrum, but a notable peak is present at 3.16 ppm in a region where there are no interfering peaks from other compounds present. Figure 3 highlights the position of this peak in relation to spectra of various coffee samples. The peak at 3.16 ppm is labeled as (i).

Figure 3. NMR spectra of 16-OMC and various coffee samples from Derfernez et al2.
Trace amounts were found to vary between samples, but these were commonly equivalent to the addition of approximately 1% of robusta to arabica - the lower limit of detection for adulteration.
The generated statistical analysis shows that arabica coffees adulterated with robusta at the 1% w/w level will be detected in around 50% of cases. This increases to 90% of cases at the 2% level, and it is doubtful that any adulterated sample will pass undetected at the 3% level.
This method's overall preparation and measurement time is 90 minutes per sample—more than four times faster than the HPLC method specified in DIN 10779. QA/QC turnaround times are greatly improved as NMR is moved out of custom-built laboratories and onto the benchtop.
Education
Education in synthetic chemistry gains significant benefit from early hands-on access to NMR, affording students a deeper understanding of this commonly used analytical technique while providing useful structural information on synthesized molecules and compounds.
The use of benchtop NMR in the laboratory speeds up data acquisition, meaning that students can analyze what they have synthesized mere minutes after the synthesis itself. Students must be well versed in NMR if they are to be prepared for a future in chemistry because this technology is employed widely throughout the biotechnology and chemical research industries.
NMR can now offer a much more hands-on experience in the educational space. For example, students learning structural elucidation are frequently provided paper copies of spectra that have been at least partially processed, sometimes with axes that are difficult to read.
Students would be required to perform the analysis, with all the information required to determine the structure already provided. This is rarely the reality in a research environment. Here, researchers almost exclusively interact with data electronically, and they may be required to identify and run more complex spectra to complete their analysis.
Thanks to benchtop NMR, these more complex spectra are readily available in the lab. Two-dimensional experiments are also routinely available in most instruments, with popular experiments such as COSY, TOCSY, and HSQC included as standard on the X-Pulse.
The extra dimension of data afforded by these experiments can help fast-track the structural determination of small molecules.
Where samples do require higher magnetic fields, benchtop NMR is a vital pre-screening tool, allowing users to confirm that the product or sample under investigation does warrant the time and cost associated with high field evaluation. This pre-screening functionality can also be beneficial for managers of NMR facilities.
Benchtop NMR requires far less space than a conventional high field set-up, also offering the potential to free up more expensive-to-run magnets and allowing these to be used more efficiently.

Figure 4. ¹H NMR spectra of 3-Dimethylaminoacrolein in CDCl₃, over a temperature range of +2 to +48 °C. Image Credit: Oxford Instruments NMR
Students utilizing the benchtop NMR variable temperature accessory will be able to follow the progression of dynamic changes in molecular structure. NMR can be used in this scenario to reinforce learning concepts from both NMR and molecular structure.
Figure 3 tracks the cis-trans isomerization of the amino-methyl groups in 3-dimethylaminoacrolein. The NMR spectra presented here highlight that the sample group signals coalesce into a single signal.
Increasing sample temperature increases the molecule’s rotational energy, speeding up the rotation of the methyl groups until these are spinning so rapidly that they become equivalent. It is then possible to calculate the thermodynamic barrier for the rotation of the carbon-nitrogen bond.
Using a benchtop instrument, NMR experiments can now follow a comparable pattern, allowing students to experience prediction, experiment, and results in the same session.

Figure 5. Image Credit: Oxford Instruments NMR
Reaction monitoring
NMR offers benefits for both research and teaching beyond structural elucidation, particularly due to its ability to easily integrate flow cells into a benchtop instrument.
Benchtop NMR allows the analysis of a reaction’s success to be run in minutes. There is no longer a need to travel to a user facility or send samples to external companies for routine analysis.
For example, the reaction shown below provides data on the flow rate and temperature-dependent esterification of ethanol and ethanoic acid catalyzed by sulphuric acid. Ethyl ethanoate is synthesized in this example - a solvent commonly used for dry cleaning, stains, varnishes, lacquers, fats, and nitrocellulose.

Figure 6. Schematic (above) and physical set-up (below) in a fume hood showing how flow chemistry is incorporated in the X-Pulse benchtop NMR instrument. Image Credit: Oxford Instruments NMR
A flow cell set-up (Figure 6) was used with the X-Pulse, and the reaction was performed at varying temperatures to investigate the rate constant dependence on temperature.
Table 2. Oxford Instruments NMR
Temperature / oC |
Temperature / K |
Rate constant / moI-1 dm3 s-1 |
22 |
295 |
2.3x10-5 |
37 |
310 |
5.1x10-5 |
50 |
323 |
1.0x10-4 |
57 |
330 |
1.7x10-4 |
72 |
345 |
3.6x10-4 |
Table 2 displays the temperature-dependent variation in the rate constant. NMR can also be used to determine enthalpy (ΔH), entropy (ΔS) (linked to reaching the transition state from the reactants), and Gibbs Free Energy (ΔG).
Conclusion
Cryogen-free, benchtop NMR has successfully enabled university teaching courses, analytical scientists, and industrial research facilities to employ the NMR technique directly in their laboratories.

Image Credit: Oxford Instruments NMR
The advent of benchtop NMR has effectively eliminated the high upfront and maintenance costs of conventional high-field NMR. The broadband X-Pulse NMR’s capacity to include comprehensive temperature and flow control allows a single instrument to meet a range of analytical needs, from student learning through to high-end research and development and industrial quality assurance/quality control.
References and further reading
- E. O. Stejskal & J. E. Tanner, J. Chem. Phys., 1965, 42, 288-292.
- M. Defernez; E. Wren; A. D. Watson; Y. Gunning; I. J. Colquhoun; G. L. Gall; D. Williamson & E. K. Kemsley, Food Chem., 2017, 216, 106-113
Acknowledgments
Produced from materials originally authored by William G. Hale, James T. Sagar, Rosie E. Jones, and Robin J. Blagg from Oxford Instruments.
About Oxford Instruments NMR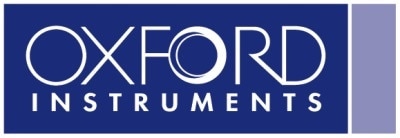
Oxford Instruments offers a range of Nuclear Magnetic Resonance (NMR) instruments to multiple industries. Our portfolio includes, X-Pulse, a high-resolution cryogen-free broadband benchtop NMR spectrometer. Combining true broadband X-nuclei capability, flow chemistry, reaction monitoring and variable temperature with superior spectral resolution, X-Pulse lets you perform a wide range of experiments on the bench in your lab.
The MQC+ range of benchtop NMR analysers offers broad applications across the agriculture, foods, consumer products, textiles, and polymer industries. MQC+ analysers are used to measure oil, water, fluorine, and solid fat in a variety of samples and are typically used for quality assurance and quality control. To increase laboratory productivity and efficiency, MQC+ analysers can be used with the MQ-Auto sample automation system. MQR is a low-resolution, high-performance TD-NMR research system designed for applications based on relaxation and/or diffusion measurements. Our GeoSpec NMR core analysers are designed specifically for studies of core samples from oilfield reservoirs, with installations in almost every major oil producer and SCAL laboratory world-wide.
Sponsored Content Policy: News-Medical.net publishes articles and related content that may be derived from sources where we have existing commercial relationships, provided such content adds value to the core editorial ethos of News-Medical.Net which is to educate and inform site visitors interested in medical research, science, medical devices and treatments.