Process development is key to identifying the optimal parameters used for synthesis, manufacturing, and stability testing. It is also key to generating the data required for the filing processes of many pharmaceutical regulatory bodies.
NMR is widely used in the structural characterization of impurities, intermediates, and final products. Cryogen-free benchtop NMR spectroscopy can also be used to explore chemical pathways and better optimize the synthesis process to accommodate the drug target molecule.
Small molecule pharmaceuticals’ synthesis processes typically involve multi-step organic chemical reactions. In circumstances where the structure of a target intermediate or the location of a characteristic NMR peak is known, there is no longer any need to completely elucidate the structure.
The simple detection and identification of characteristic peaks can quickly determine the presence of expected intermediates and products in these intermediate process reactions, saving users time, work, and money.

Figure 1. One-dimensional 1H stacked spectra of a reaction mixture before and after reaction. Image Credit: Oxford Instruments NMR
Two one-dimensional 1H spectra of a compound prior to (orange spectrum) and following (blue spectrum) the synthesis process is shown in Figure 1. These spectra were each acquired with an approximately one-minute acquisition time, clearly demonstrating sufficient signal-to-noise to make key decisions about the synthesis process.
It is noteworthy that the two 1H spectra’s overall peak shapes are very similar, particularly in the 0-5 ppm region. Differing chemical shifts and splitting patterns shown in the characteristic peaks in the 7-9 ppm region confirm that the chemical reaction has altered the compound, however.
It would be possible to determine whether the product in this example is an expected intermediate or a different compound by leveraging further spectral analysis via database matching or comparison with predicted spectra. This could be done by also evaluating information about the relevant reactant structures, reaction mechanism, and process flow.
For example, fluorine is a high-sensitivity NMR active nucleus frequently found to be more powerful for reaction monitoring than the more widely used hydrogen. Thanks to the less complex fluorine NMR spectra and the wider chemical shift dispersion, straightforward resolution of reactant and product signals is possible.
It is possible to use 19F NMR in this instance to provide key information for a diverse array of pharmaceuticals, including a number of new APIs containing fluorine. Two stacked 19F spectra acquired prior to (orange spectrum) and following (blue spectrum) a synthetic process are displayed in Figure 2.

Figure 2. One-dimensional ¹⁹F NMR spectra acquired before (lower) and after completion of (upper) a reaction (spectra unreferenced). Image Credit: Oxford Instruments NMR
The change is clear in this example. A new peak appears in the spectrum following the reaction, highlighting the addition of another type of fluorinated group to the molecule. The X-Pulse can quickly provide screening results in any standard laboratory, due to its capacity to offer these straightforward one-dimensional spectra.
Knowing the identities of reactants and products is essential in drug development and production, as is understanding the reaction process and kinetics.
A benchtop X-Pulse NMR can be equipped to study and monitor reactions using a flow chemistry module directly connected to an external reaction vessel. This setup allows the reaction mixture to be continuously pumped through the flow cell, allowing chemical reactions to be monitored online and in real time.
The pharmaceutical industry widely uses 3-nitrobenzaldehyde, an important intermediate in the synthesis of Nitrendipine, Nimodipine, and Nicardipine.
Using a sodium borohydride catalyst, 3-nitrobenzaldehyde can be reduced to 3-nitrobenzyl alcohol. The flow rate can be continuously pumped into the X-Pulse NMR flow cell every 20 seconds, and a 1H spectrum can be recorded.

Figure 3. Stacked series of one-dimensional 1H NMR spectra acquired during online monitoring of the reduction of 3-nitrobenzaldehyde to 3-nitrobenzyl alcohol. Image Credit: Oxford Instruments NMR
Results of online chemical reaction monitoring using a one-dimensional 1H spectrum are shown in Figure 3. The reaction mixture in this example was pumped continuously through the flow cell at a rate of 1 ml/minute, and a spectrum was collected every 20 seconds.
The aldehyde group of the reactant (located at around 10 ppm) was found to entirely disappear in the tenth spectrum. This spectrum was acquired approximately 200 seconds after the reaction had been initiated, confirming the rapidity of the reaction process.
It was also noted that the resolution of the benzene ring region located between around 7-9 ppm was found to suddenly deteriorate in the ninth spectrum. This is likely due to the formation of an intermediate product with a medium rate of exchange on the NMR timescale.
Later spectra continued to lack the aldehyde peak while exhibiting a higher-resolution benzene ring region. This likely indicates that the reaction had been completed.
Benchtop NMR is used to monitor chemical reactions online. This allows the concentration curves of reactants and products to be obtained over time. Using this data, the order and rate constant of the chemical reaction can be determined.
Acquiring chemical reaction rate constants under various temperature conditions enables the calculation of enthalpy, entropy and Gibbs free energy changes, as well as other kinetic reaction parameters.
This data is especially valuable for improving reaction efficiency, optimizing reaction conditions, controlling the reaction process, and better designing reactors.
Acknowledgments
Produced from materials originally authored by Oxford Instruments.
About Oxford Instruments NMR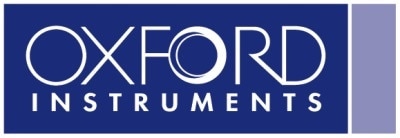
Oxford Instruments offers a range of Nuclear Magnetic Resonance (NMR) instruments to multiple industries. Our portfolio includes, X-Pulse, a high-resolution cryogen-free broadband benchtop NMR spectrometer. Combining true broadband X-nuclei capability, flow chemistry, reaction monitoring and variable temperature with superior spectral resolution, X-Pulse lets you perform a wide range of experiments on the bench in your lab.
The MQC+ range of benchtop NMR analysers offers broad applications across the agriculture, foods, consumer products, textiles, and polymer industries. MQC+ analysers are used to measure oil, water, fluorine, and solid fat in a variety of samples and are typically used for quality assurance and quality control. To increase laboratory productivity and efficiency, MQC+ analysers can be used with the MQ-Auto sample automation system. MQR is a low-resolution, high-performance TD-NMR research system designed for applications based on relaxation and/or diffusion measurements. Our GeoSpec NMR core analysers are designed specifically for studies of core samples from oilfield reservoirs, with installations in almost every major oil producer and SCAL laboratory world-wide.
Sponsored Content Policy: News-Medical.net publishes articles and related content that may be derived from sources where we have existing commercial relationships, provided such content adds value to the core editorial ethos of News-Medical.Net which is to educate and inform site visitors interested in medical research, science, medical devices and treatments.