Many functions have been attributed to extracellular vesicles (EV), due to their rich content of mRNA/miRNA, ADAM protease enzymatic activity, surface receptors and cytokines, chemokines, and other soluble factors (CCF). Now it appears that DC-derived EV are able to differentiate monocytes
After it was found that MHC class-II-enriched vesicles could bring about antigen-specific T-cell responses, dendritic cell (DC)-derived EV were studied at an early stage.
These studies indicated that DC-derived EV have a number of functions in immune regulation and can modulate T-cell responses by interacting with DC, as well as T cells. Further to their antigen-presenting abilities, it was discovered that DC-derived EV activated NK cells and could, through the presence of TNF, FasL, and TRAIL, destroy tumor cells.
Aside from typical or classical hematopoietic stem cell–derived DC (cDC1/cDC2) and plasmacytoid DC (pDC), monocyte-derived cell populations exert antigen-presenting immune functions. Moreover, an increasing number of researchers agree that humans monocytes give rise to inflammatory DC [(inf)DC], which are distinguishable by the expression of a set of markers (HLA-DR, CD11c, BDCA1, CD1a, FcεRI, CD206, CD172a, CD14, CD11b, and Zbtb46).
While the functions of these cells are comparable to those of conventional DC, their transcriptome is distinctive and they are thought of as inflammatory monocyte-derived cells. As such, they may inhabit the far end of a multipurpose and condition-adaptable monocyte cell population.
The earlier noted human 6-sulfo LacNAc (slan)–expressing DC are believed to have originated from blood CD16+ monocytes and are also categorized into this inflammatory cell type. In further support of this judgment, slanDC were identified in the tissue of chronic inflammatory diseases, including psoriasis and lupus erythematodes.
There is emerging agreement that, just as with humans, inflammatory DC in mice also originate from monocytes. An inflamed environment appears to be a particular catalyst for this, as shown in a variety of mouse inflammation models.
Adding weight to this conclusion, the quantity of developing (inf)DC is not affected in Flt3L−/− mice, while cDC, which rely on Flt3L stimulation, are drastically diminished. On the other hand, in mice deleted for the monocyte migration marker CCR2, (inf)DC are significantly reduced in inflamed tissue.
In the first instance, mouse monocyte–derived (inf)DC were determined to be positive for MHC-II, CD11b, CD11c, F4-80, and Ly6C, and further markers have since been discovered, including FcεRI and CD64. Nevertheless, the immediate precursors of these cells are not adequately characterized, and the method of development of these monocyte-derived DC precursors remains ambiguous.
Both generally accepted beliefs and the wealth of studies carried out thus far would indicate that this is dependent not only on the inflammatory cytokine milieu, but also on further parameters, including tissue-specific and possibly host-specific factors.
Early studies have demonstrated that monocytes are effective at ingesting EV. Within this article, it is shown that DC-derived EV, as a result of their rich content of cytokines, chemokines, and soluble ligands, can mobilize and distinguish monocytes into an assortment of phenotypes, which it is probable will intensify and adapt an inflammatory immune response to a particular situation.
This study highlights EV’s significance in the function and initiation of the immune cell network.
Skin-Injected BMDC-EV Attract Immune Cells
PKH-labeled imBMDC-EV and maBMDC-EV, were injected into the skin of mice in order to imitate the secretion of EV from DC in vivo. The skin patches surrounding the injection sites, alongside the draining lymph nodes, were gathered 6 hours and 24 hours following injection, demonstrating a red color (Fig 1).
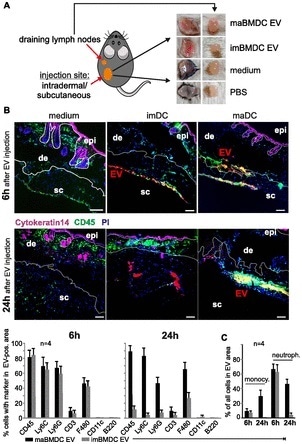
Figure 1. Skin-injected BMDC-EV attract immune cells. (A) Cartoon depicting the injection site of PKH26-labeled BMDC-EV, and images from excised skin patches and draining lymph nodes used for MELC analysis and marker quantification. For control, PKH26-containing medium and PBS were injected. (B) BMDC-derived EV attract immune cells in the skin. Tissue sections from skin patches described in (A) were subjected to a MELC analysis. Images represent an overlay of four markers (CD45, cytokeratin-14, PI, and EV). Cytokeratin-14 and CD45 were stained by antibodies, whereas EV (red stain) were visualized through PKH26. Tissue sections from two animals (6 h and 24 h) are presented. Using the StrataQuest software, the relative presence (in percentage) of common immune cell markers was quantified in EV-containing tissue areas. The relative presence by a bar diagram as indicated. Note: individual images for these markers are presented in Fig 5. Quantifications of MELC analyses from four different injection sites were used to determine the SEM. Scale bars represent 100 μm. (C) Monocytes and neutrophils in imDC-EV and maDC-EV areas. Monocytes (CD11b+/Ly6C+/Ly6G−) and neutrophils (CD45+/Ly6C+/Ly6G+) were quantified in the EV areas using the StrataQuest software as explained in Table 1 and Fig S8A. epi, epidermis; de, dermis; sc, subcutaneous.
The multiepitope ligand cartography (MELC) technology, which was recently shown to allow sequential staining of the same tissue section by multiple antibodies, was employed to examine portions of these tissues using immunofluorescence.
Furthermore, improved imaging software (StrataQuest from TissueGnostics) with the capacity to quantify multiple immunofluorescence markers in tissue (Fig 2) was also utilized.
Through the combination of these dual methods of analysis, it was possible not only to identify, but to quantify a number of markers in tissue areas containing EV. Sections of tissue containing EV were defined by the software, as outlined in Fig 2a.
Confocal images were taken from tissue sections indicating EV granules in the cytoplasm and perinuclear region of CD11b+ cells Fig 2b in order to corroborate that the injected EV had been ingested by cells rather than simply deposited.
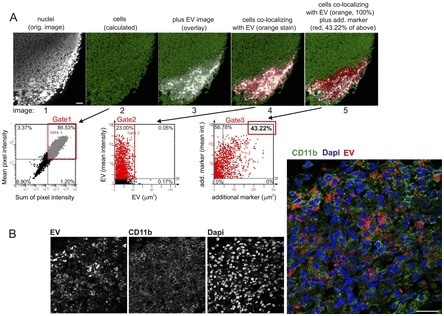
Figure 2. Marker identification and quantification by StrataQuest, and confocal analysis of EV-containing tissue sections. (A) StrataQuest analysis algorithm. First, nuclei are identified and quantified (gated) through propidium iodide (PI) assessment (gated nuclei: 86.53%; first two images and gate 1). Around the nuclei, the software calculates and demarcates a small area that represents the cell cytoplasm and boundary (green net-like structure in image 2). Subsequently, a marker image is superimposed, demarcating the area of interest (here area of EV deposition in a lymph node, images 3 and 4, gate 2), which is set to 100% in order to calculate cell sub-populations in this area. Subsequently, an additional marker is superimposed and gated on the previous gate (43.22% of cells in the EV area, gate 3). This calculation can be performed for different individual markers, or continued for assessing multiple marker co-expression in one cell. Scale bars represent 100 μm. (B) Analysis of cellular EV uptake in tissue. A tissue section maDC-EV 6 h after injection was analyzed by confocal microscopy in order to demonstrate that EV were ingested by cells and not merely deposited by injection. To indicate the cytoplasm, the cells were stained for CD11b. EV are indicated by red color and nuclei by DAPI. Scale bars represent 25 μm.
In addition to control injections (PKH26 and PBS), four of these regions (examples in Fig 3) were examined for each time point (6 h/24 h) and each EV type (EV from im-/maDC) in four different animals.
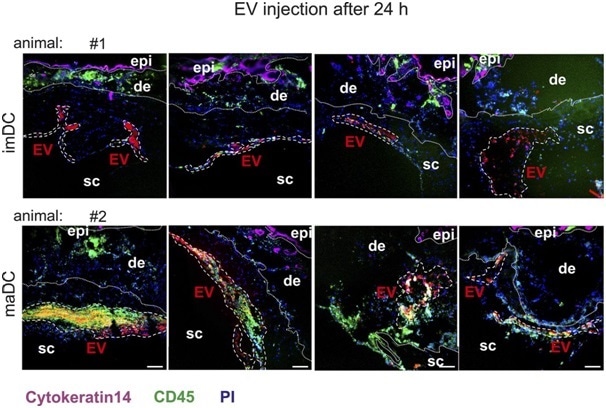
Figure 3. Representative examples of EV injection sites. Shown are examples of im- and maBMDC-EV injection sites after 24 h in two different animals (four skin injections per animal). The EV areas are demarcated with white interrupted lines. For each time point and each EV type (immature/mature), one animal with four injection sites was analyzed. Scale bars represent 100 μm.
Discussion
It can be seen that EV shed by maDC stimulate the differentiation of monocytes towards imDC in vitro and possibly in vivo, as indicated by the development of DC-typical functional properties and surface marker expression.
This differentiation mechanism was dependent on effector molecules present in these vesicles, including GM-CSF, which is typically needed for the differentiation of monocytes into DC in vitro.
Nonetheless, the abundance of effectors identified in maDC-EV indicated more intricate functions and target cell effects, and blocking trials with anti-GM-CSF may have restricted the internalization process of EV altogether.
The injection of murine BMDC-EV into mouse skin uncovered at least one of these functions. The vesicles attracted mainly myeloid cells and to some extent T cells, possibly through chemokines such as MCP-1 and TARC.
Acknowledgments
This article was produced from materials originally found at: http://www.life-science-alliance.org/content/1/6/e201800093. This article is available under a Creative Commons License (Attribution 4.0 International, as described at https://creativecommons.org/licenses/by/4.0/).
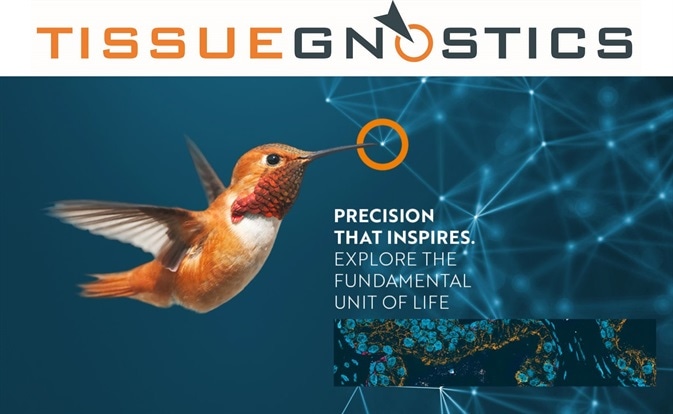
About TissueGnostics
TissueGnostics (TG) is an Austrian company focusing on integrated solutions for high content and/or high throughput scanning and analysis of biomedical, veterinary, natural sciences and technical microscopy samples.
TG was founded by scientists from the Vienna University Hospital (AKH) in 2003. It is now a globally active company with subsidiaries in the EU, the USA and China and customers in 28 countries.
TG systems offer integrated workflows, i.e. scan and analysis, for digital slides or images of tissue sections, Tissue Microarrays (TMA), cell culture monolayers, smears and of other samples on slides and oversized slides, in Microtiter plates, Petri dishes and specialized sample containers.
Sponsored Content Policy: News-Medical.net publishes articles and related content that may be derived from sources where we have existing commercial relationships, provided such content adds value to the core editorial ethos of News-Medical.Net which is to educate and inform site visitors interested in medical research, science, medical devices and treatments.