Envisioning pathological processes in the central nervous system using preclinical MRI Magnetic resonance imaging (MRI) is a well-known means of imaging for clinically managing patients with neurological conditions. It offers both macro- and microstructural tissue markers and, therefore, provides critical guidance for making clinical decisions and predicting outcomes, together with neurological evaluations.
MRI is a key tool for drug development and conducting research on patients with neurological conditions. As the quantity of experimental and transgenic animal models of human neurological conditions grows, MRI has become a valuable instrument in preclinical neurological research.
The animal models represent vascular, inflammatory, neurodegenerative, traumatic, ischemic, and neoplastic processes, and preclinical MRI has been utilized to define the effects of such cellular changes on nervous tissue integrity. Furthermore, preclinical MRI supports drug discovery and development and validates clinical imaging findings, contributing to a growing understanding of human neurological conditions.
Dedicated small animal MRI tools have been built to image mice, rats, and other model organisms. Bruker's BioSpec Maxwell series combines the most recent developments in preclinical MRI technology.
These Maxwell magnets, which do not need liquid cryogen filling, are available at 3 Tesla, 7 Tesla, and 9.4 Tesla field strengths (Fig. 1), all with 17 cm bore diameter, and are fitted with high-performance gradient systems (gradient strength up to 900 mT/m, slew rate = 4200 T/m/s). The three-field strengths are suitable for various needs, facilitating translational studies at 3 Tesla and 7 Tesla, and taking advantage of heightened signal-to-noise ratios at 9.4 Tesla.
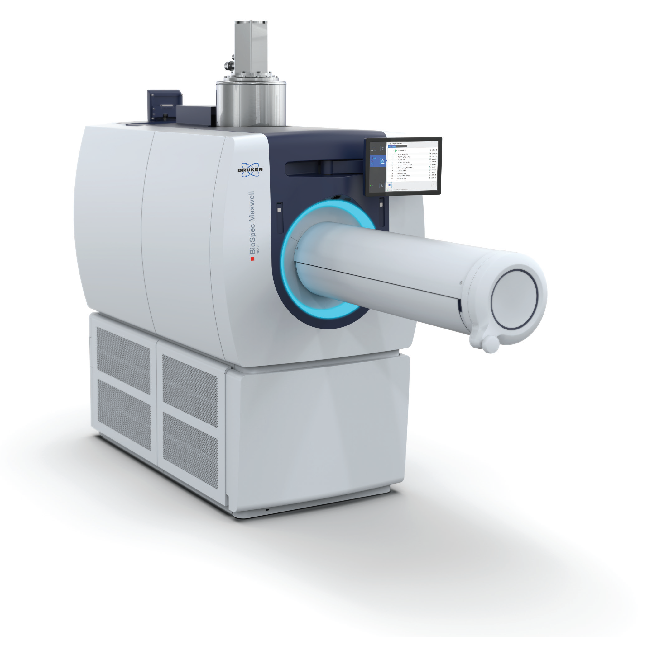
Figure 1. BioSpec Maxwell. BioSpec Maxwell MRIs are compact liquid cryogen filling-free MRI instruments available at field strengths of 3 Tesla, 7 Tesla, and 9.4 Tesla. Image Credit: Bruker BioSpin - NMR, EPR and Imaging
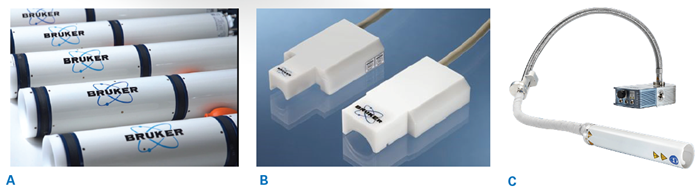
Figure 2. BioSpec Maxwell portfolio of dedicated RF coils. A) With up to 82 mm inner diameter, the range of volume coils for BioSpec Maxwell instruments accommodates preclinical imaging species ranging from mice up to large rats. B) Dedicated brain and spine coils are optimized to ideally match anatomical regions of interest. C) For studies requiring highest resolution, the MRI CryoProbe provides a sensitivity boost. Image Credit: Bruker BioSpin - NMR, EPR and Imaging
BioSpec Maxwell tools can be used in conjunction with volume and surface coils, which are well-suited for imaging the brain and spinal cord (Fig. 2A, B). Cryogenic surface coils enable significant increases in sensitivity that may be invested for achieving enhanced spatial resolution or for reducing the scans’ acquisition time (Fig. 2C).
Bruker’s ParaVision offers a software framework for MR method development and execution of pre-optimized protocols for common animal species and use cases. The software suite comes with the planning and acquisition of scans, and its viewing, reconstruction, and analysis instruments deliver the most information from gathered imaging data.
MRI acquisition protocols like T1-weighted or T2-weighted imaging can offer rich anatomical contrast and provide data on the location and extent of a central nervous system injury. These sequences can capture morphological alterations in the brain and spinal cord both acutely and over time.
Other acquisition protocols can evaluate specific tissues in the brain and spinal cord. For instance, time-of-flight angiography can envision large blood vessels and, therefore, identify pathological aberrations in vasculature. Recent research has concentrated on developing sophisticated MRI methods that yield metabolic and functional data from the central nervous system.
These readouts deliver more accurate assessments of central nervous system damage. As metabolic and functional differences often come before changes in tissue morphology, they may also constitute more sensitive markers of disease.
Monitoring disturbances in energy metabolism and neurotransmitter systems
Metabolic disturbances are a widespread feature of neurodegenerative and inherited conditions, and tumors and are a key focus area of preclinical neurological research. The preferred method for imaging metabolism in the central nervous system is positron emission tomography (PET). PET tracers such as [18F]-Fluorodeoxyglucose and [15O]-oxygen are utilized to quantify the regional cerebral metabolic rate of glucose and oxygen metabolism.
Bruker’s BioSpec Maxwell MR tools can be used in conjunction with a PET inline or PET insert module for sequential or simultaneous PET/MR imaging, respectively, and therefore utilized to evaluate metabolic processes in preclinical models (Fig. 3). Moreover, numerous MRI approaches measure metabolic processes in the brain and spinal cord.
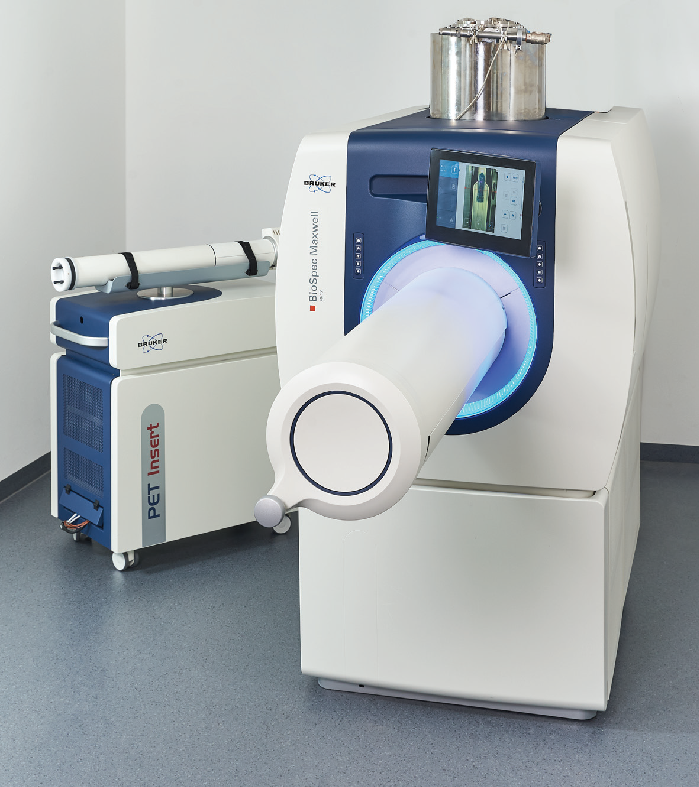
Figure 3. Multi-modal PET/MR imaging. PET inline and insert modules are available for sequential or simultaneous PET/MR imaging, enabling multi-parametric investigations. Image Credit: Bruker BioSpin - NMR, EPR and Imaging
Magnetic resonance spectroscopy (MRS) uses common approaches for quantifying regional biochemistry in the central nervous system.1 The most utilized methods are localized 1H MRS acquisition approaches that offer a range of neurotransmitters and metabolites in an area.
Fig. 4A depicts two examples of localized 1H MR spectra of the mouse brain obtained using a BioSpec Maxwell 30/17 tool with a transceive cryogenic quadrature radiofrequency surface probe (MRI CryoProbe) and a BioSpec Maxwell 94/17 tool with a linearly polarized bird-cage resonator for transmission and a phased-array receive-only surface coil in cross-coil mode.
The use of the MRI CryoProbe helps visualize small molecule resonances even at 3 Tesla, yet MRS at 9.4 Tesla yields a 1H spectrum with elevated spectral separation and signal-to-noise ratio. The gains result in significantly more detectable metabolites.
MRS imaging of several voxels enables the assessment of neurotransmitter and metabolite concentration variability in diverse areas. Fig. 4B demonstrates chemical shift imaging of the mouse brain captured using a BioSpec Maxwell 94/17.
Localized 1H MRS and MR spectroscopic imaging can evaluate regional variations in cell density, cell type, and metabolite concentrations and distinguish healthy tissues from pathological tissues. For instance, it can identify lesions of various underlying pathologies that have a similar appearance in structural MRI.
The use of higher-field MR tools, dynamic acquisition approaches, and advanced quantification approaches produces functional MRS data with high temporal resolution, which enables the assessment of pathology-related dynamic changes in neurotransmitters in predefined areas.
Moreover, X-nuclei (e.g. 2H, 17O and 31P) MRS imaging approaches additionally benefit from usage of higher field strengths regarding sensitivity. For instance, in deuterium (2 H) metabolic imaging, 2H MRS imaging is carried out following the oral intake or intravenous infusion of 2H-labeled substrates and maps of cerebral glucose metabolic rates are produced.
Glucose metabolism dysfunction in neurodegenerative conditions is linked to altered tissue function and viability, while in contrast, tumor growth is linked to higher glucose metabolism.
Other quantitative and noninvasive assessments via X-nuclei MRI are used in cerebral metabolic rates of oxygen consumption and adenosine triphosphate production, alongside the assessment of intracellular nicotinamide adenine dinucleotide metabolites and redox state.
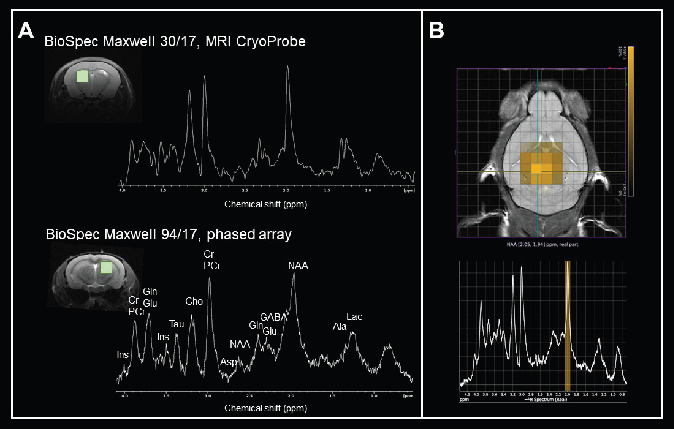
Figure 4. Monitoring levels of neurotransmitters and metabolites. A) Axial FLASH images of the mouse brain with volume of interest and in vivo 1H MRS spectra measured from the corresponding brain regions at 3 Tesla and at 9.4 Tesla. Localization sequence: PRESS, volumes 2 x 2 x 2 mm3. The following metabolites were assigned: alanine (Ala), aspartate (Asp), choline (Cho), creatine (Cr), γ-aminobutyric acid (GABA), glutamate (Glu), glutamine (Gln), myo-inositol (Ins), lactate (Lac), N-Acetylaspartate (NAA), phosphocreatine (PCr), and taurine (Tau). Data was acquired with a BioSpec Maxwell 30/17 equipped with a transceive cryogenic quadrature radiofrequency surface probe (MRI CryoProbe) and a BioSpec Maxwell 94/17 using a linearly polarized bird-cage resonator for transmission and a phased-array receive-only surface coil in cross-coil mode. Spectra were processed with TopSpin using the same parameters: line broadening 2, Fourier transform and phase correction. B) Chemical shift imaging of the mouse brain. The morphological T2-weighted TurboRARE image shows the position of the CSI grid with a voxel size of 1.25 x 1.25 x 2 mm3. The corresponding 1H MRS-spectra from the selected voxel are displayed beneath. Data was acquired with a BioSpec Maxwell 94/17 using a linearly polarized bird-cage resonator for transmission and a phased-array receive-only surface coil in cross-coil mode. Image Credit: Bruker BioSpin - NMR, EPR and Imaging
Another approach to MRS is chemical exchange saturation transfer (CEST) MRI. In comparison to MRS, CEST MRI can identify biochemical molecules of interest with both more sensitivity and more spatial resolution. In CEST MRI, a radiofrequency pulse is applied to a molecule of interest, which can exchange its 1H protons with those of water at one or more of its resonant frequencies to achieve a saturation state.
This magnetic saturation is spontaneously transferred to the water via the chemical exchange of the excited metabolite protons with non-excited water protons. The inevitable decrease in water signal can then be identified using standard MRI sequences, providing an indirect assessment of the molecule of interest’s concentration.
Known endogenous CEST molecules usually contain exchangeable groups of amide protons (protein and peptides), amine protons (e.g. glutamate and creatine), and hydroxyl protons (e.g. glycosaminoglycan, myo-inositol). It is possible to use them to study variations in the concentrations of metabolites and neurotransmitters in neurological conditions.
Based on metabolic signatures, CEST MRI can be used to grade brain tumors and evaluate tumor therapies. Moreover, some exogenously administered compounds can be used as CEST agents. These include glucose and certain drugs which allow the study of cerebral and tumor glucose metabolism to track drug actions.
Assessing altered neurofluid patterns and metabolic waste removal
Neuronal metabolic activity generates potential toxic by-products. To preserve homeostasis, metabolic waste should be cleared rapidly from the central nervous system. Critical routes for clearing waste include interstitial and cerebrospinal fluids that transport metabolic solutes.
For instance, true fast imaging with steady-state precession (TrueFISP) sequence contrasts cerebrospinal fluid spaces (Fig. 5A) and 3D renderings (Fig. 5B), allowing the assessment of ventricle anatomy and morphology, a critical area for cerebrospinal fluid production and transport.
Disrupted ventricles are seen in numerous congenital neurological conditions and in response to neuronal injury or degeneration. Contrast-enhanced, flow and diffusion MRI approaches facilitate the monitoring of interstitial and cerebrospinal fluid flow through various extracellular compartments in the brain and spinal cord.
A number of neurological conditions exhibit changes in the magnitude and direction of cerebrospinal fluid flow, which are linked to impaired waste removal, accumulation of metabolites (e.g., lactate, ß-amyloid), and trafficking of immune cells. Assessing neuro fluid flow patterns with MRI can aid the assessment of different therapies to see whether they can restore normal fluid passage.
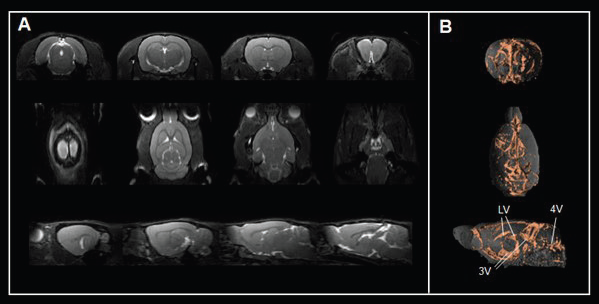
Figure 5. Assessing brain ventricles. A) Axial, coronal, and sagittal T2-weighted TrueFISP images and B) corresponding renderings reveal the cerebrospinal fluid spaces in the rat brain. Rat brain data was acquired with a BioSpec Maxwell 94/17 using a volume coil for transmission and a phased-array coil for reception. Tissues were segmented and rendered using PMOD. LV = lateral ventricle; 3V = third ventricle; 4V = forth ventricle. Image Credit: Bruker BioSpin - NMR, EPR and Imaging
Probing vascular dysfunction
The central nervous system is critically dependent on a continuous supply of oxygen and nutrients to properly function. Failure in substrate supply will disturb homeostasis and incur tissue damage, alongside functional loss. Vascular dysfunction (e.g. occlusion, hemorrhage, calcifications) leads to neurological deficits in cerebrovascular conditions and in neurodegenerative conditions, where vascular alterations can be seen in causal pathways.
Various preclinical MRI approaches exist that can probe vasculature’s functional properties.2 Quantitative data about cerebral blood flow (CBF) and cerebral blood volume can be gained through arterial spin labeling, dynamic susceptibility contrast MRI, vascular space occupancy and other MRI approaches.
An example of a regional CBF (rCBF) map of a mouse brain obtained from a pulsed arterial spin labeling approach is displayed in Fig. 6A. The map demonstrates variations in rCBF in various anatomical areas of the brain (cortex = 125.7 ± 36.4 ml/100g/min, thalamus = 204.1 ± 80.5 ml/100g/min). Combining perfusion approaches with pharmacological vasodilatory stimuli enables the assessment of vessel vascular reactivity.
Dynamic contrast-enhanced MRI can measure changes in the blood-brain barrier integrity, where an impairment is linked to an influx of intravascular compounds from the blood to the brain. Vascular MRI approaches are used to assess the effects of aging, diet, and environmental factors on vascular function and how this translates into cognitive deficiency and tissue damage.
Vascular dysfunction can be investigated in suitable disease models and when evaluating therapeutics for vascular diseases. Furthermore, MRI can deliver insights into the cardiovascular system (e.g., cardiac function, aortic stiffness) and, therefore, enable investigation of how vascular risk factors impact central nervous system function.
Mapping dysfunction of functional networks and neuronal plasticity
Functional magnetic resonance imaging (fMRI) is utilized to track changes in neuronal activity in both the brain and spinal cord. In fMRI, temporal and spatial alterations in the blood oxygen level-dependent (BOLD) signal are recorded, typically with a T2*-weighted gradient echo planar imaging (EPI) sequence.
Fig. 6B shows an example of EPI images of the mouse brain obtained with a BioSpec Maxwell 94/17. In a task-based fMRI experiment, BOLD signals are assessed in response to a stimulus (sensory, thermal, acoustic, pain, or other) to detect areas that are functionally implemented in the performance of specific tasks.
In resting-state functional MRI, BOLD data is gathered in task-free states. The measured low-frequency fluctuations enable the evaluation of regional interactions, which are used to outline specific functional networks in the brain and spinal cord and/or to generate a functional connectome that enables a global assessment of both circuitry and circuit function.
Animals are typically anesthetized during assessment. However, fMRI in awake but immobilized mice and rats is becoming more common, reducing the confounding effects of anesthesia and allowing the study of animals during behavioral tasks.
For pixelwise analysis of signal variations, scanner performance stability is key. An excellent time course stability of repeated EPI acquisitions could be shown via a BioSpec Maxwell 94/17 (Fig. 6C). Drift compensations are applied via additional navigator signals that are obtained and utilized to apply magnetic field corrections to the working frequency, reaching a temporal signal-to-noise ratio = 123).
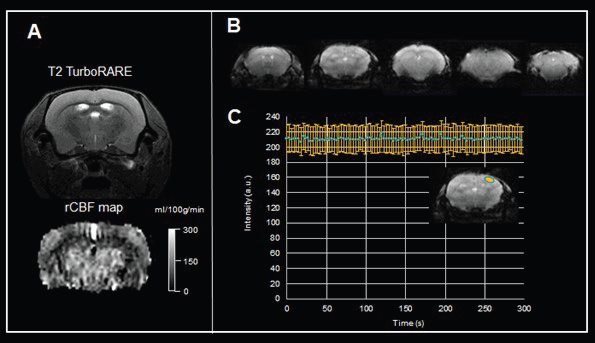
Figure 6. Functional MRI of the brain. A) Pulsed arterial spin labeling of the mouse brain. Shown are a T2-weighted TurboRARE image for anatomical reference, and the rCBF map computed from the flow-sensitive alternating inversion recovery (FAIR)-EPI method. Data were acquired with a BioSpec Maxwell 94/17 using a linearly polarized bird-cage resonator for transmission and a phased-array receive-only surface coil in cross-coil mode under isoflurane anesthesia. B) Example of gradient echo EPI images of the mouse brain acquired with a BioSpec Maxwell 94/17 equipped with a 23 mm volume coil. Shown are five 0.6 mm thick axial slices with an in-plane resolution of 141 μm x 156 μm. C) The time course characteristics of EPI imaging for fMRI studies were assessed by acquiring 100 repetitions of gradient echo EPI over an acquisition time of 5 minutes with active drift compensation. Acquisition shows solid time course stability with a temporal signal-to-noise ratio = 123. Image Credit: Bruker BioSpin - NMR, EPR and Imaging
Functional MRI is utilized to evaluate central nervous system dysfunction on the regional or network level during the development of neurodegenerative conditions. It also enables the study of plasticity and functional recovery of the brain and spinal cord following acute injury.
Resting state functional MRI, together with behavioral tests, allows the construction of computational models to link symptoms to changes in circuit function.
A key constraint of BOLD-fMRI is that it only enables the indirect measure of neuronal activity. The BOLD contrast is regulated by neurovascular coupling, which can be changed during development, aging, disease conditions, and anesthesia.
Therefore, an observed change in BOLD signal may not be linked to disrupted neuronal activity if neurovascular coupling is compromised. Nevertheless, a distinct advantage of preclinical fMRI research is that it can be merged with pharmacological, chemo- and optogenetics, or electrical neuromodulation methods and/or invasive readouts (electrophysiology, optical imaging, etc.).
These methods can then be utilized to determine the underlying cellular mechanisms of the observed fMRI read-outs or to carry out mechanistic or interventional research.3 Furthermore, attempts are made to track neuronal activity directly with fMRI, heightening spatial and temporal precision.
Conclusion
Preclinical MRI and MRS approaches enable sensitive and specific assessments of metabolic and functional processes in the central nervous system that occur early on in the development of neurological conditions. The approaches have become crucial instruments in preclinical neurological research for understanding the underpinnings of various diseases, for drug discovery and development, and for providing disease biomarkers.
References and further reading
- Lanz, B. et al. (2020). Magnetic resonance spectroscopy in the rodent brain: Experts’ consensus recommendations, NMR in Biomedicine, 34(5). https://doi.org/10.1002/nbm.4325.
- Klohs, J. (2019). An Integrated view on vascular dysfunction in Alzheimer’s Disease, Neurodegenerative Diseases, 19(3–4), pp. 109–127. https://doi.org/10.1159/000505625.
- Lee, J.H., Liu, Q. and Dadgar-Kiani, E. (2022). Solving brain circuit function and dysfunction with computational modeling and optogenetic fMRI, Science, 378(6619), pp. 493–499. https://doi.org/10.1126/science.abq3868.
About Bruker BioSpin - NMR, EPR and Imaging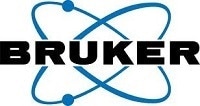
Welcome to Bruker BioSpin and the world's most comprehensive range of NMR and EPR spectroscopy and preclinical imaging research tools. The Bruker BioSpin Group of companies develop, manufacture and supply technology to research establishments, commercial enterprises and multi-national corporations across countless industries and fields of expertise.
Bruker BioSpin is continuing to revolutionize the design, manufacture and distribution of life science, preclinical, process control and analytical research tools based on magnetic resonance and multimodal imaging technologies. Bruker BioSpin is the worldwide technology and market leader in magnetic resonance technologies (NMR, EPR) and offers the largest portfolio of imaging modalities for preclinical and industrial research under a single brand.
Sponsored Content Policy: News-Medical.net publishes articles and related content that may be derived from sources where we have existing commercial relationships, provided such content adds value to the core editorial ethos of News-Medical.Net which is to educate and inform site visitors interested in medical research, science, medical devices and treatments.