This article outlines improvements and changes to non‐viable particle monitoring (NVP), sometimes referred to as total particulate monitoring, which during aseptic processing, is a regulatory requirement.
Aseptic processing is becoming more automated and more crucial to future products of the biopharmaceutical industry. Similarly, NVP monitoring is also becoming more automated and more crucial for contamination risk management during aseptic processing.
Growth in aseptic processing
Vaccine production has historically made up a big part of aseptic production. Recent years have seen growing focus on new biologic therapies, most of which are administered via IV or injection.
In order to drive the development and release of these new biologics, major pharmaceutical companies are investing. As most of these will be produced with aseptic processing, the following trends should be considered:
- Biologics are expected to comprise half of the top 100 selling drugs by 20161
- The molecular complexity of biologics means each product can have specific delivery needs1 (i.e. specific sterile packaging, like injection technologies or custom syringes)
- Over a thousand biologics are currently either approved or in clinical development1
- As sales grow quickly for biologics and vaccines sales, growth for small molecule drugs (i.e. pills) is slowing2
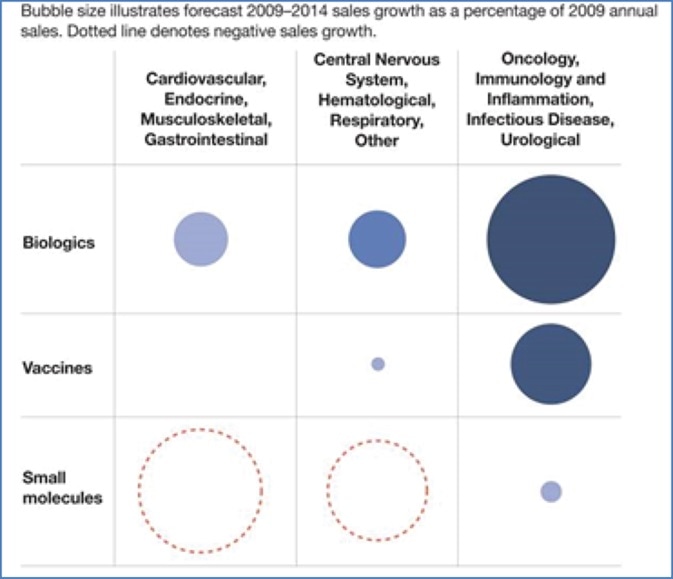
Image Credit: Beckman Coulter Life Sciences
Components for a number of biologic therapies come from cell line production activities. Gene therapies are developed and produced with certain cell lines needing aseptic processing.
In the developing markets, vaccine production continues to grow, also through new vaccine-based therapies, for example new cancer prevention treatments (e.g. HPV). New biologic therapies have arrived, or are in the approval process, for the following conditions:
- Oncological
- Urological
- Cardiovascular
- Inflammation
- Immune system
- Hematological
- Musculoskeletal
- Gastrointestinal
- Central nervous system
- Respiratory
The rate of approval for biologics is a lot higher than it is for new small molecule treatments. Investment is also increasing in biosimilars, due to the profitability of these new biologic therapies.
All of these factors highlight the growing need for aseptic processing into the foreseeable future. A lot of these aseptic processing facilities will require custom handling and packaging equipment, together with more use of automation.
Aseptic processing improvements and the need for event detection
It is widely accepted that the leading cause of microbiological contamination in aseptic processing is human contact with sterile products and components.
There is an increased use of automated equipment, isolators and Restricted Access Barrier Systems (RABS) as new aseptic processing facilities are built, in order to combat this and reduce human contact with sterile products.
The contamination control priority is reducing human contact, along with improving unidirectional filtered (HEPA) air flow coverage, such as 100% HEPA coverage for exposed sterile products and components.3
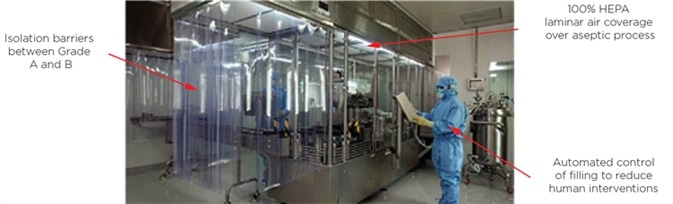
Figure 1. Modern aseptic liquid fill operation. Image Credit: Beckman Coulter Life Sciences
The greatest single advancement in aseptic processing has been the elimination of interventions using various types of automation. Examples include, depyrogenation tunnels, automatic lyophilizer loading, clean-in-place/sterilize-in-place systems and automated weight check/adjustment.3
From Akers’ and Allacogo’s article, “Clean Rooms, RABS and Isolators: Validation and Monitoring in the Diverse World of Aseptic Processing”, These aseptic technology improvements have started to appear in regulatory guidance, like the EU GMP requirement, beginning in 2011, to extend continuous Grade A air flows over stoppered, but uncapped, containers.
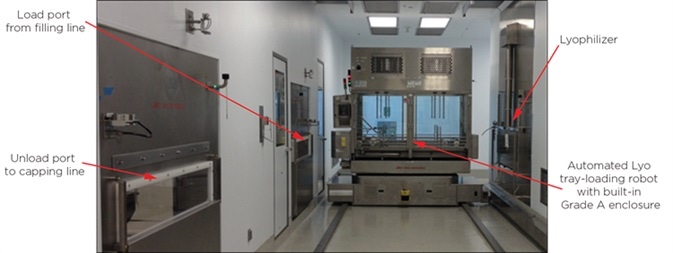
Figure 2. Modern isolated aseptic lyophilization operation. Image Credit: Beckman Coulter Life Sciences
The virtual elimination of viable counts during processing is the result of these improvements in modern manufacturing environments. It is now challenging, if not impossible, to determine a statistically meaningful baseline of viable count levels during processing activities.
Increasing monitoring intensity (i.e. viable EM [environmental monitoring]) in nearly all instances is only going to result in more zeroes, a phenomenon we’ve seen over and over again. Too often the most common intervention in aseptic processing is environmental monitoring, which is completely illogical. What this means is that the method erroneously relied upon to measure risk is itself the greatest source of human contamination risk!3
The focus of contamination monitoring has now moved from daily processing activities to uncommon events during processing due to isolation and improved laminar airflows, these include momentary disruptions of the environment or equipment, failure to follow SOPs, human interventions, etc.
Event detection requires continuous sampling and non-zero results during events. Real-time event alarms are important to limit the exposure of sterile products to events. Everything is getting cleaner; the risks are now less consistent and more random.
The role of non-viable particle monitoring
As this is the critical metric for optimizing and validating aseptic environments and processes during media fills, the emphasis in aseptic process monitoring is on viable particle EM. Viable particle monitoring during production supplies a reference to the original validated aseptic conditions which were determined during media fill studies.
Most particles produced in the daily operation of modern aseptic environments are sterile foreign material. These submicron particles are not detected by viable particle EM techniques.
Viable samples from operations usually produce a series of zero counts (CFU’s) and these samples only “spot check” aseptic conditions because of their infrequent (non-continuous) nature. Although this viable sampling is a recommendation of regulators, it supplies no immediate information to capture environmental events.
When properly configured, continuous non-viable particle monitoring (NVP), gives useful information on changes in the aseptic environment with minute-by-minute updates of count populations and trends, which catch contamination events and so, identify and quantify risks (i.e. a non-zero result).
Biological contamination may be a possibility if root cause investigations for these non-viable particle events identify human involvement in the event. Non-biological foreign material contamination may be a possibility If the event was caused by automated equipment or components.
So, assurance of minimum risk begins with continuous non-viable particle monitoring. Although viable particle EM is crucial, especially to qualify aseptic environments, it is an indicator of risk which is less reliable during sterile product processing, and it does not identify the risk of non-biological foreign matter contamination.
Foreign matter testing in liquid sterile products (via USP 788 testing of injectables) tests a small amount of final products (the product is consumed during testing).
This is crucial to qualify the process for large particle contamination from the process and packaging, but during an event in a large batch, it is unlikely to catch foreign matter affecting a small number of products at risk.
It is the combination of all test techniques: non-viable, viable, and USP, which produces an umbrella of protection during the production of sterile products. Each test technique adds a layer of protection, but it is the unique role of non-viable air sampling to detect real-time changes and events that could compromise portions of aseptic lots. "Where advanced aseptic technologies are used we should be relying almost exclusively on evaluation of physical parameters, and electronic particulate monitoring, which produce data that can be reviewed in real time rather than requiring several days of incubation." 3 Akers and Allacogo
We believe that in advanced aseptic systems electronic total particulate analysis is largely sufficient to provide the information required to ensure the maintenance of a validated state of environmental control. If a microbiological sampling component is retained by regulators it should operate at a much lower sampling intensity than that currently expected in manned clean rooms.3
Akers and Allacogo
Setting NVP alarms to capture particle events
Regulatory guidance recommends monitoring non-viable particle counts in two size ranges: particles 0.5 micron and larger, and particles 5.0 micron and larger. The EU recommends monitoring both size ranges and the FDA focuses on the 0.5 micron size range.
Continuous monitoring of NVP during aseptic processing for the purpose of event detection is referenced by both the EU and FDA.
|
At Rest |
In Operation |
Grade |
Maximum permitted number of particles/m3 equal to or greater than the tabulated size |
|
0.5 µm |
5 µm |
0.5 µm |
5 µm |
A |
3,520 |
20 |
3,520 |
20 |
B |
3,520 |
29 |
352,000 |
2,900 |
C |
352,000 |
2,900 |
3,520,000 |
29,000 |
D |
3,520,000 |
29,000 |
not defined |
not defined |
Figure 3. EU Annex 11 Particle Limits (Tighter than ISO 14644 standard for 5 um in Grade A/B). Image Credit: Beckman Coulter Life Sciences
“The Grade A zone should be monitored at such a frequency and with suitable sample size that all interventions, transient events and any system deterioration would be captured and alarms triggered if alert limits are exceeded.” 2009 EU Annex 11. This statement brings about a requirement for continuous monitoring and alarms.
In order to quickly capture and alarm on particle events, NVP monitoring systems update once per minute. Both the 0.5 and 5.0 micron size ranges are monitored every minute, and count results are generated for a cubic meter air volume, gathered over the last 36 minutes, and a cubic foot air volume, which is gathered over the last minute.
The cubic foot volume is helpful for event detection; the cubic meter volume is a comparable reference to the ISO cleanroom standard (ISO 14644) and EU non-viable count limits.
Setting alarm limits for 5.0 micron particles
A lot has been written about how 5.0 micron and bigger particles can be a food source for bacteria and how most bacteria are detected in this size range. Yet, particles that are 5.0 micron and larger are a lot less mobile in air than the smaller 0.5 micron particles. Therefore, the 5.0 micron particles are a lot harder to detect.
It should be understood that airborne particle counters all require the particle to pass through the instrument to be detected and so particle “transport” and “suspension” are crucial. Micron particles of 5.0 are rare in modern isolators and RABS, even during events.
Per cubic meter, 20 particles is the regulatory limit (EU Annex 11) for 5.0 micron in Grade A, which is under one particle per minute in real-time NVP monitoring systems (e.g. 36 one-minute samples = one cubic meter of air).
A count alarm limit set at one count for a single sample is not statistically significant but many systems are set for Action alarms on one 5.0 micron particle because of the EU limit. Ironically, some viable particle limits will accept one CFU per sample.
A better Action alarm limit for NVP would be to alarm on three consecutive one-minute samples of one particle at 5.0 micron. This shows a particle production source which is significant.
Any inquiry into root cause should carefully consider if the event involved a human intervention. It is also worth noting that mechanical failures due to friction, like a bad bearing on an automated robot, usually produce large particles.
Setting alarm limits for 0.5 micron particles
Typically, particle generation sources create more small particles than large ones, and small particles are easier to gather and transport in an airstream. So, it is at smaller sizes that a particle generation event is more likely to be detected.
A particle event that does not register 5.0 micron particle counts, but shows 0.5 particles, may still generate particles of a larger size. The larger particles may not be counted as there are fewer of them and they do not travel as well in the air sample. So, in NVP monitoring, more attention should be paid to the smaller 0.5 micron particles.
A common misconception is that Action alarm limits for 0.5 micron may also be set at the Grade A limit, which is 3,520 particles per cubic meter. This translates to an Action limit of 98 particles per minute (i.e 1 cubic foot sample).
This particle level is a lot higher than the average particle level for modern aseptic processing environments that are in operation (i.e. not “at rest”), which can average somewhere between zero and 10 particles per minute of the 0.5 micron size.
At a certain sample location, if an aseptic process is averaging 5 particles per minute, a sample which jumps to 90 particles is an increase of 18 times – but it may not trip an Action alarm. Such a huge increase shows a particle generation event; which is an event that may have also produced larger particles which may not have been detected.
The best way to set 0.5 micron NVP count alarm levels is to employ the same practices that were utilized for setting viable count limits in older environments containing humans:
- Set the limit to capture events which are statistically significant deviations from the baseline
- Utilize non-viable particle data from the media fill to establish the baseline count level
To summarize, employ both of these principles to identify potential NVP contamination events during aseptic processing (i.e. “in operation” alarm limits):
- Based on the results of the media fill study, highlight 0.5 micron count alarms and set the limit low enough to identify deviations from the normal count baseline.
- Set the 5.0 micron Action alarm levels to identify multiple consecutive samples with single counts or large one-sample events.
Modern NVP monitoring systems
As aseptic processing environments are improving the non-viable monitoring systems which are utilized to monitor them are improving too. The below trends are starting to appear in NVP monitoring of aseptic processes:
- Increase in the number of particle sensors, especially in automated fill/finish operations. Modern aseptic lines, particularly those which are involved in lyophilization, have many robotic devices.
Two or three filling lines may provide a common bank of lyophilizers. Automation includes automatic vial weighing devices, mobile Grade A containment systems for moving partially stoppered product to lyophilizers, automatic tray loading devices, lyophilizer loading robots, automatic stopper inspection/rejection systems, etc.
An operation such as this expands the size of the ISO5 Grade A and B areas, with many points for human intervention into isolators to service automation equipment during an event.
Sterile components and products are exposed to more equipment (but less human contact) and move further distances. A risk analysis of potential contamination events generates more points which must be continuously monitored in this type of workflow.
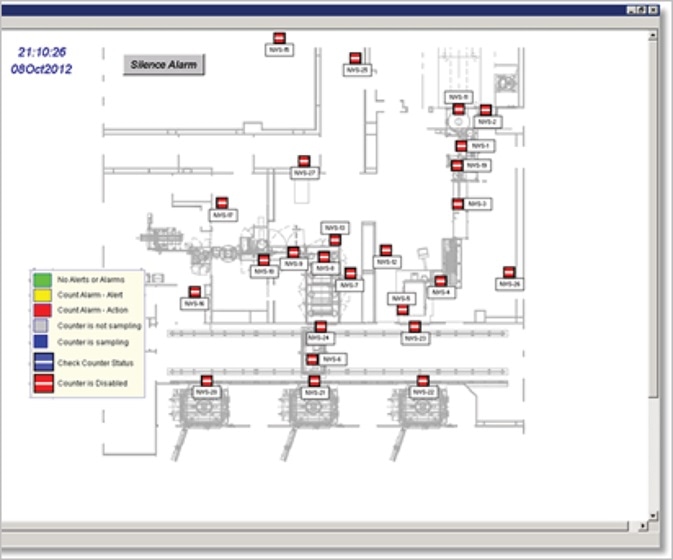
Figure 5. Screen capture from monitoring system for Lyo batch operation. (22 sensors controlled in three groups: Filling, Lyo Loading, and Capping). Image Credit: Beckman Coulter Life Sciences
- More regulatory focus on decreasing the employment of human-operated, portable, particle counters for online, permanently installed, computer-controlled particle counters.
- Reduces need for operators in Grade B areas
- Enhances the consistency of monitoring to SOPs
- Decreases the requirement for operator presence in Grade A to place probes and take samples
- Removes operators from managing data (e.g. manual data uploads, paper tapes, etc.) to decrease collection or transcription errors
- Removes equipment from aseptic Grade B (i.e. large carts/portables) which can interfere with airflow and introduce additional surface areas to the sterile core
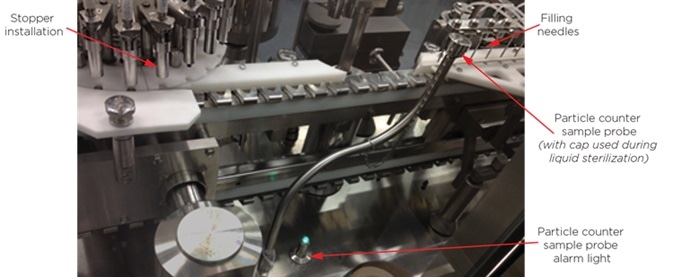
Figure 4. Permanent installation of online particle counter for continuous sampling during filling. Sensor is installed outside of Grade A/B – inside the filling equipment. Image Credit: Beckman Coulter Life Sciences
- The utilization of recipe-driven monitoring systems based on workflow. Modern particle monitoring systems control sensors based on the workflow state.
Monitoring software prequalifies the aseptic environment for At Rest cleanliness levels and prequalifies sensor cleanliness (zero counting) before exposing sterile product.
Throughout product processing, the right sensors are monitored based on which stage of the operation is active (e.g. capping, filling). During Action level alarms, until aseptic conditions are reviewed and approved, equipment is automatically paused.
- Alarm notification and acknowledgment which captures the root cause at the time of the event. Operators are now required to identify the event associated with the particle event in modern systems. This information is stored and automatically and included in batch reports for review and approval.
The batch reports also reveal non-viable particle trends, which help to establish whether an event is the result of slowly-increasing particle levels which eventually tripped an Alert or Action level alarm, or a short duration, isolated event that is not statistically significant.
- Batch-driven operation and reporting for non-viable/viable particle monitoring. Particle monitoring systems now automatically label data with batch/lot identifications and produce comprehensive batch reports immediately after the aseptic process is finished.
These reports include statistics, sample data, and alarm histories that have assignable causes. Sample data is recorded for quality purposes if production is halted to carry out an intervention, but as long as sterile product was not exposed during the intervention this data may be excluded from batch reports.
Viable samples can also be controlled from the non-viable monitoring system, operator per plate, including air sample volumes, sample plate start/stop times, flow alarms, and resamples, with all data recorded to ensure a total viable/non viable EM program for the batch.
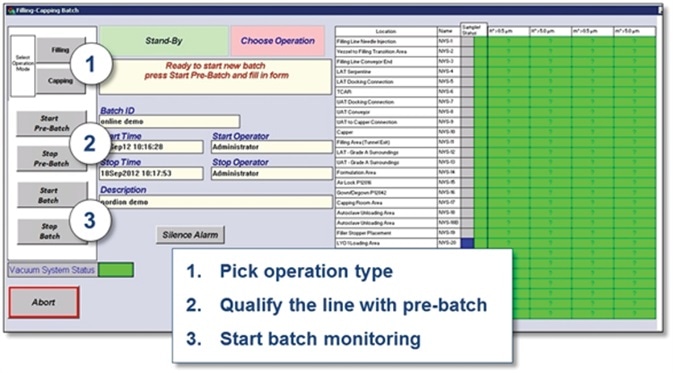
Figure 6. Screen capture showing batch monitoring process. (The right sensors and alarm limits are automatically used for each process step. Results are reported per batch.). Image Credit: Beckman Coulter Life Sciences
Modern continuous NVP monitoring systems generate the data reports and alarm history automatically in order to ensure continuous control of aseptic environments so that the product batches can be released safely.
Secondarily, in order to drive continuous reduction of risks to sterile products, the comprehensive nature of the monitoring allows the meaningful analysis of particle events.
Benefits of NVP monitoring in modern aseptic areas
The careful implementation of non-viable particle monitoring uniquely offers the below benefits for automated and isolated aseptic areas as the biopharmaceutical industry becomes more dependent on aseptic processing for new therapies:
- Detects all particles at defined risk points
- Detects smaller particles which are more populous during events
- Detects both viable & non-viable (foreign material) particles
- Supplies useful non-zero data during events
- Continuously monitors to capture all events
- Does not need human intervention to sample isolated Grade A areas
- Can automate monitoring and batch report generation to save labor and decrease errors
- Supplies immediate results for real-time alarms to protect sterile product
Summary
Modern non-viable particle monitoring systems are able to supply assurance of aseptic processing whilst decreasing human involvement in aseptic areas for monitoring purposes.
These systems are able to supply data to simplify root cause alarm investigations, enhance aseptic conditions over time, and generate instant compliance reports for batch release – removing the labor associated with creating reports.
References
- Excerpts from Unilife’s description of biologic drugs. http://www.unilife.com/blog/biologic-drugs
- Summary of sales data from National Center for Biotechnology Information, a reprinted article from Biotechnology Healthcare: With Injectable Biologic Therapies on the Rise, Payers Face Tough Reimbursement Issues. http://www.ncbi.nlm.nih.gov/pmc/articles/PMC2899801/
- Quotes from article on American Pharmaceutical Review: Clean Rooms, RABS and Isolators: Validation and Monitoring in the Diverse World of Aseptic Processing. http://www.americanpharmaceuticalreview.com/Featured-Articles/36878-Clean-Rooms-RABS-and-Isolators-Validation-and-Monitoring-in-the-Diverse-World-of-Aseptic-Processing/
About Beckman Coulter Life Sciences
Beckman Coulter Life Sciences is dedicated to empowering discovery and scientific breakthroughs. The company’s global leadership and world-class service and support delivers sophisticated instrument systems, reagents and services to life science researchers in academic and commercial laboratories, enabling new discoveries in biology-based research and development.
A leader in centrifugation and flow cytometry, Beckman Coulter has long been an innovator in particle characterization and laboratory automation, and its products are used at the forefront of important areas of investigation, including genomics and proteomics.
Primary activity / Product lines
- Flow Cytometry
- Centrifugation
- Particle Counting and Characterization
- Liquid Handling and Robotics
- Nucleic Acid Sample Preparation
Sponsored Content Policy: News-Medical.net publishes articles and related content that may be derived from sources where we have existing commercial relationships, provided such content adds value to the core editorial ethos of News-Medical.Net which is to educate and inform site visitors interested in medical research, science, medical devices and treatments.