The adult human heart ventricle consists of cardiomyocytes, fibroblasts, endothelial cells, and other types of supporting cells. Despite cardiomyocytes being 75% of the total volume of the human ventricle, it only comprises 50% of the total number of cells.
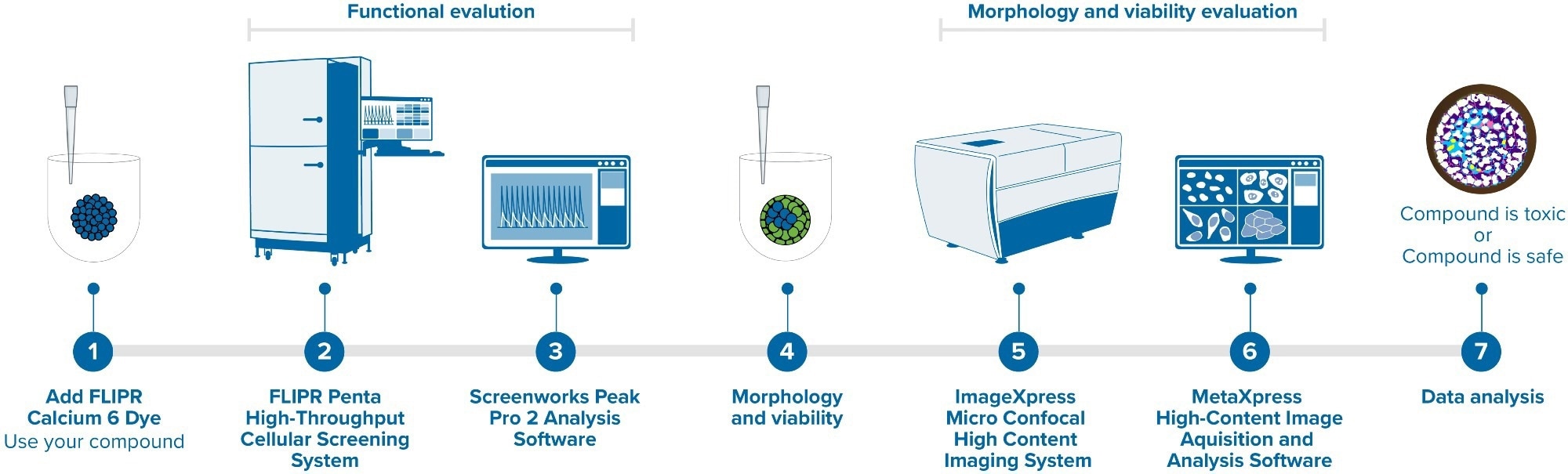
Image Credit: Molecular Devices UK Ltd
Recent publications have demonstrated that tri-cellular co-culture microtissues of cardiomyocytes, cardiac fibroblasts, and endothelial cells (all from human iPSC) improve the functional activity and maturation of cells compared to 2D cardiomyocytes. As a result, they tend to more closely mimic genuine heart physiology.
This article discusses the utilization of a tri-culture model produced by mixing iPSC-derived cardiac cells and endothelial cells with primary adult fibroblasts in the ratio of 75:15:10 in ultra-low attachment (ULA) plates directly from the thaw.
Benefits
- The tri-culture model provides a novel tool for the evaluation of functional and morphological effects on cardiac cells.
- The FLIPR Penta System ensures better resolution of calcium oscillation patterns in cardiomyocytes.
- MetaXpress CME allows individual cells to be located and 3D structures of cell models to be reconstructed.
A Biomek i7 Automated Workstation (Beckman Coulter Life Sciences) was employed for cell plating with later media exchange every two days. Within 48 hours, 3D microtissues were formed, which started to contract spontaneously and regularly on day five.
The functional activity of microtissues was studied by recording calcium oscillations after the addition of calcium dye using the fast kinetic fluorescence recording instrument, FLIPR® Penta High-Throughput Cellular Screening System, as displayed in Figure 1.
Figure 1. FLIPR Penta High-Throughput Cellular Screening System and ImageXpress Micro Confocal High-Content Imaging System. Image Credit: Molecular Devices UK Ltd
The response of the microtissues to several known modulators of cardiac activity was tested. It was found that molecules such as isoproterenol significantly increased the rate of oscillation and the peak amplitude (inotropic response).
Other compounds, such as hERG inhibitors, ion channel blockers, or beta-blockers, exhibited changes in the Ca2+ oscillation patterns as expected. The ScreenWorks® Peak Pro 2™ software module was employed to perform waveform analysis.
High-content imaging was utilized to characterize the structure and morphology of the 3D microtissues with the ImageXpress® Micro Confocal High-Content Imaging System, as shown in Figure 1.
Different cell types were immunostained using antibodies specific for Troponin T (for cardiomyocytes), COL1A1 (for fibroblasts), and VE-Cadherin (for endothelial cells). The microtissues’ 3D structure was reconstructed and analyzed with the MetaXpress® High-Content Image Acquisition and Analysis Software.
The data presented in this article emphasizes the utility and biological relevance of employing iPSC-derived cell types in 3D micro-tissues as a valuable model for the measurement of compound effects on human cardiac tissues in a high-throughput format.
Instruments
The FLIPR Penta High-Throughput Cellular Screening System was equipped with a high-speed EMCCD camera for measuring the patterns and frequencies of the Ca2+ oscillations of cardiac tri-culture microtissues. These were monitored by changes in intracellular Ca2+ levels with the EarlyTox™ Cardiotoxicity Kit (Molecular Devices).
The system also utilized ScreenWorks PeakPro2 peak analysis software to enable the analysis and characterization of both the primary and secondary peaks, as well as complex oscillation patterns.
The ImageXpress Micro Confocal HighContent Imaging System was used together with a spinning disk confocal and an sCMOS camera to obtain the 3D structures of the whole microtissues, stained with antibodies in various fluorescent channels.
The custom module editor, which can be customized for adequate image analysis, was used to reconstruct the 3D structure of the microtissues.
Methods
Cryopreserved human iPSC-derived iCell® Cardiomyocytes2 and iCell Endothelial Cells were acquired from FUJIFILM Cellular Dynamics, Inc., while Primary Human Cardiac Fibroblasts Cells were acquired from Promocell.
These compounds were seeded in the ratio of 75:15:10, respectively. The time point of the workflow is shown in Figure 2.
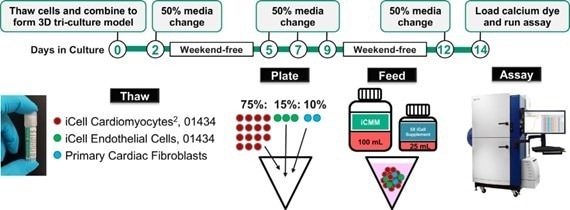
Figure 2. The workflow of the iPSC-derived Cardiac Tri-Culture Model. Image Credit: Molecular Devices UK Ltd
Before the experiments were run, the presence of strong synchronous contractions in the 3D cultures was visually confirmed.
To assess phenotypic changes, cells were stained live using a mixture of two dyes: the viability dye Calcein AM (1 μM) and the Hoechst nuclear dye (2 μM) (all from Life Technologies).
Following the compound treatment, calcium oscillations in the cells were measured using the FLIPR Penta instrument and subsequently fixed and stained using anti-Troponin T A647 with either anti-VE-Cadherin A568 or anti-COL1A1 A568.
Results
Recording and analyzing kinetic patterns
Cardiac tri-culture microtissues were loaded with the EarlyTox Cardiotoxicity Kit on the day of assay and subsequently treated with compounds for 60 minutes. Spontaneous calcium oscillations were recorded with 30–50 frames per second to enable adequate resolution of the complex oscillation patterns.
Multi-parametric characterization of the Ca2+ flux oscillation patterns was conducted using advanced analysis methods. This phenotypic assay facilitates the characterization of data, including oscillation frequency, amplitude, peak rise and decay times, peak width, and irregularity.
The appearance of early-afterdepolarization (EAD)-like event patterns, peak irregularity, and peak prolongation was also assessed. Figure 3 presents the representative tracing curves of the fluorescence signals.
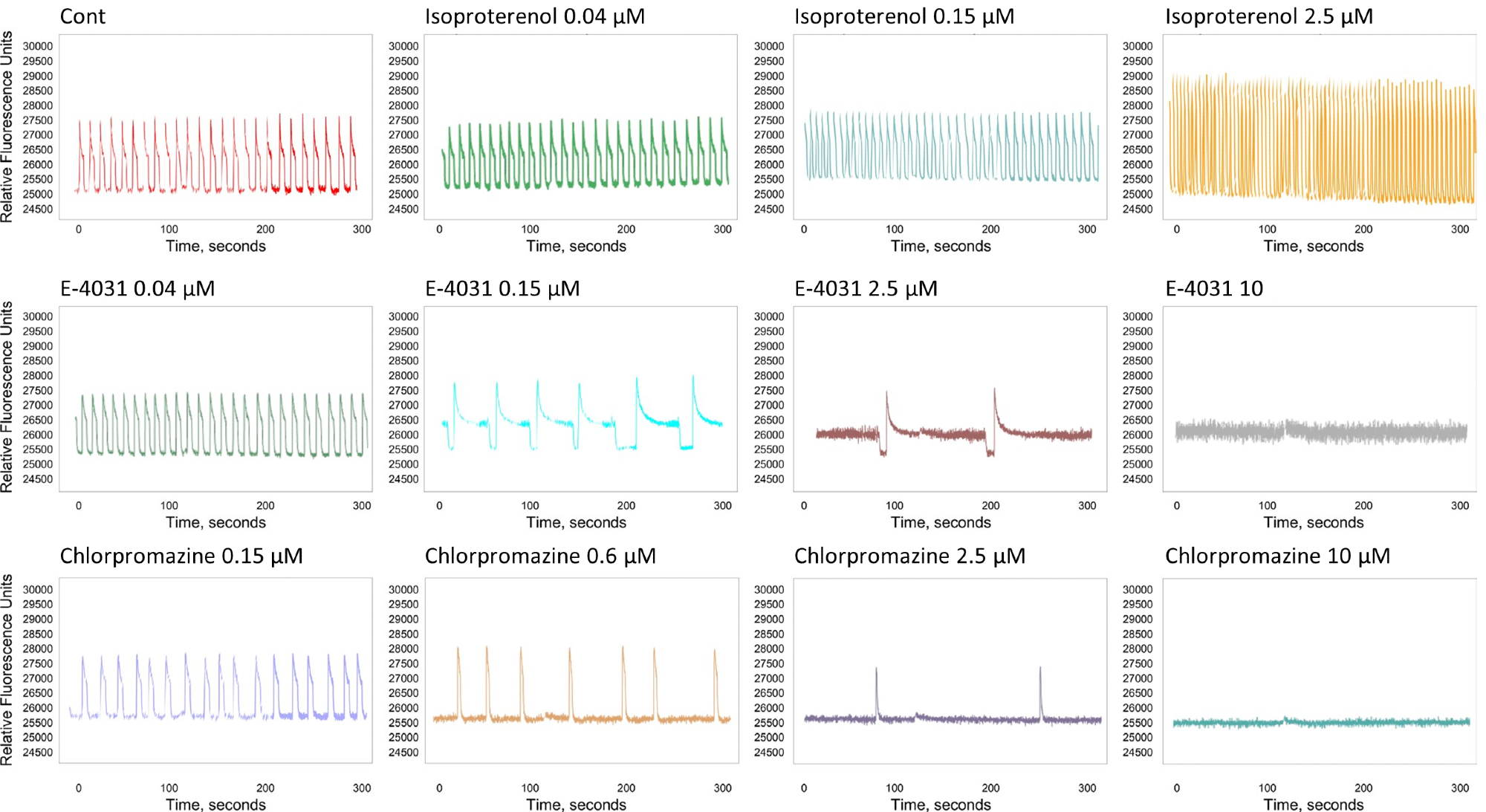
Figure 3. The tracing curve of fluorescence signals of Isoproterenol at 0.04, 0.15, and 2.5 μM; E-4031 at 0.04, 0.15, 2.5, and 10 μM; and Chlorpromazine at 0.15, 0.6, 2.5, and 10 μM. Image Credit: Molecular Devices UK Ltd
Summary of parametric effects of compounds
Figure 4 presents a summary of the trend of effects of eight modulators on the cardiac activity of microtissues, where parameters such as peak counts/300 seconds, CTD 90 (seconds), and amplitude (RFU) are included.
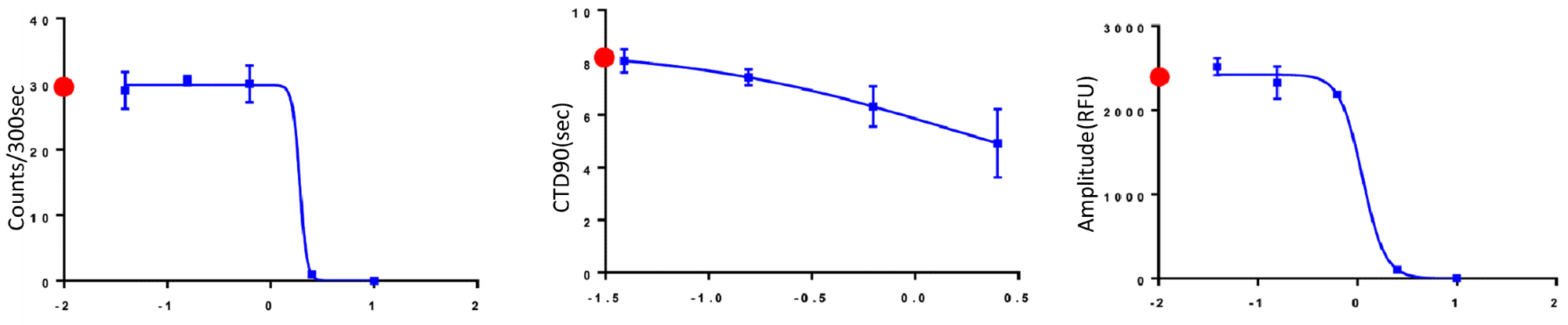
Figure 4. The trend of the change of Peak Counts/300 sec, CTD90 and Amplitude with the increasing concentration of compounds. Red dots indicated control values for appropriate measurements. Image Credit: Molecular Devices UK Ltd
As expected, well-known agonists such as isoproterenol increase the beat rate and decrease CTD90.
Other antagonists, such as chlorpromazine and cisapride, decrease the beat rate and amplitude following a specific concentration threshold. Table 1 presents a summary of the EC50 values of the compound concentration.
Table 1. Summary chart of EC50 values (µM) calculated with GraphPad Prism. Source: Molecular Devices UK Ltd
Compound |
Peak Count per 300 sec |
CTD90 (width of peak/sec at 90% of height) |
Amplitude |
MOA |
Isoproterenol |
1.25 |
0.35 |
12.76* |
B2 adrenoreceptor agonist |
Cisapride |
1.9* |
1.4 |
1.1 |
hERG blocker, antacid |
*denotes an ambiguous value in Prism.
Studying 3D cardiac microtissue structures using high-content imaging
High-content imaging of the microtissues was taken using Cy5, TRITC, and DAPI channels, with several z-planes captured to cover the whole microtissue. The captured images were subsequently analyzed using MetaXpress’ built-in custom module editor for the analysis of the 3D coordinates of each cell.
Figure 5 displays the representative overlay images of the combination of cardiomyocytes and endothelial cell staining and the combination of cardiomyocytes and fibroblast cell staining.
The images imply that the cardiac fibroblast cells are inclined to create clustering structures compared to that of endothelial cells. A silhouette analysis was conducted to study this occurrence further.
3D reconstruction of cell distribution and silhouette analysis of clustering
A silhouette score was utilized to investigate the clustering of the cardiac fibroblast cells, which ranged from -1 to +1, to measure the distance between each point in one cluster and the points in the neighboring clusters. Here, +1 denotes that the point is far from the neighboring clusters.
The 3D reconstruction of the fibroblast cells in Figure 5A is shown in Figure 5C, where 4 clusters are obvious and gain the highest silhouette score (data not shown).
The overall silhouette analysis of fibroblast cells shows that the fibroblast cells have a higher tendency to form clusters, though further research is still needed to determine whether this tendency helps the maturation of cardiac microtissues or not.
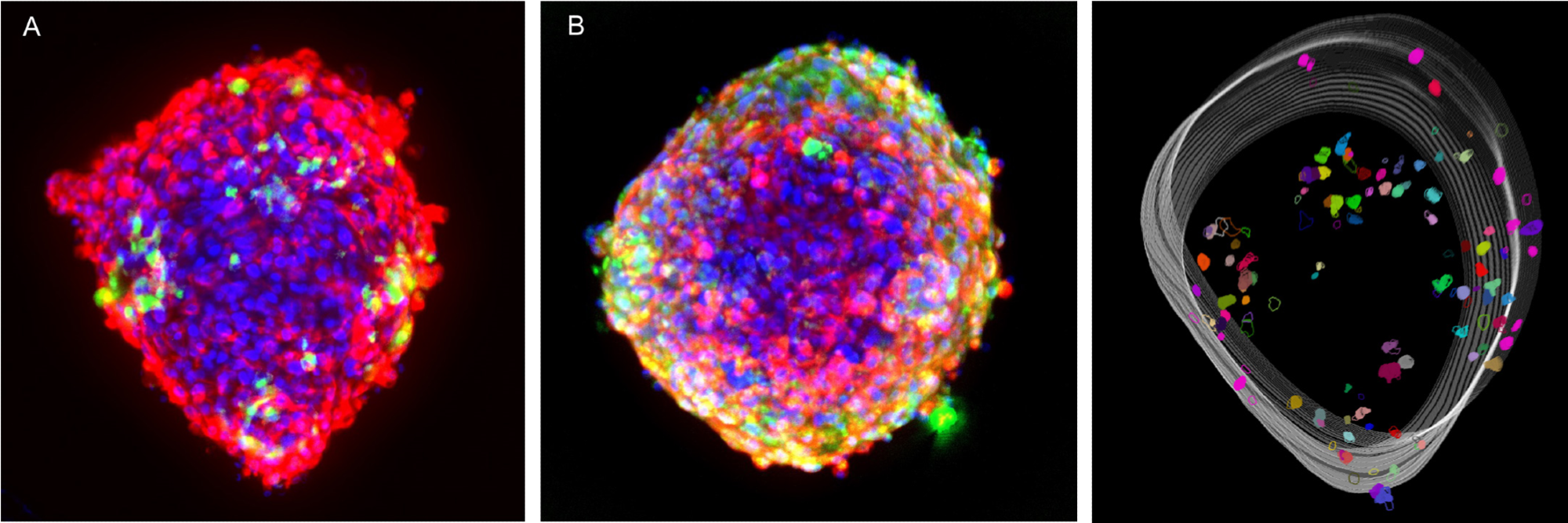
Figure 5. Composite images of cardiac tri-culture microtissues. After fixation and permeabilization, cells were stained either with a combination of anti-Troponin T AF647 + anti-VE-Cadherin AF568 or anti-Troponin T AF647 + anti-COL1A1 AF568. Cells were imaged with the DAPI, TRITC and Cy5 channels, 10X Plan Fluor objective. (A) Red: iCell Cardiomyocytes, Green: Cardiac Fibroblast Cells, Blue: Hoechst; (B) Red: iCell Cardiomyocytes, Green: iCell Endothelial Cells, Blue: Hoechst; (C) 3D reconstruction of fibroblast cells from the microtissues shown in Figure 5A. Image Credit: Molecular Devices UK Ltd
Conclusions
- Tri-culture models were generated by mixing iPSC-derived iCell Cardiomyocytes2 with iPSC-derived iCell Endothelial Cells and primary adult fibroblasts. These models deliver a novel tool for the evaluation of morphological and functional effects on cardiac cells.
- Using the FLIPR Penta System with a new high-speed camera enables increased resolution of calcium oscillation patterns in cardiomyocytes.
- The ImageXpress Micro Confocal high-content imaging system facilitated the fast acquisition of complex biological samples with high resolution.
- The MetaXpress custom module editor is a powerful tool for analysis, allowing individual cells to be located and the 3D structure of complex cell models to be reconstructed.
- A set of eight known modulators of cardiac activities showed various parametric responses.
- The assay can be used to test developing drugs and screening chemicals for potential cardiotoxic hazards.
About Molecular Devices UK Ltd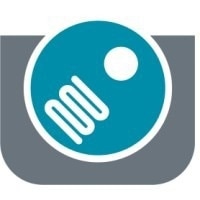
Molecular Devices is one of the world’s leading providers of high-performance life science technology. We make advanced scientific discovery possible for academia, pharma, and biotech customers with platforms for high-throughput screening, genomic and cellular analysis, colony selection and microplate detection. From cancer to COVID-19, we've contributed to scientific breakthroughs described in over 230,000 peer-reviewed publications.
Over 160,000 of our innovative solutions are incorporated into laboratories worldwide, enabling scientists to improve productivity and effectiveness – ultimately accelerating research and the development of new therapeutics. Molecular Devices is headquartered in Silicon Valley, Calif., with best-in-class teams around the globe. Over 1,000 associates are guided by our diverse leadership team and female president that prioritize a culture of collaboration, engagement, diversity, and inclusion.
To learn more about how Molecular Devices helps fast-track scientific discovery, visit www.moleculardevices.com.
Sponsored Content Policy: News-Medical.net publishes articles and related content that may be derived from sources where we have existing commercial relationships, provided such content adds value to the core editorial ethos of News-Medical.Net which is to educate and inform site visitors interested in medical research, science, medical devices and treatments.