Lungs are highly vulnerable to injury and infection due to continuous exposure to inhaled environmental pathogens and particles. As a result, respiratory diseases are a leading cause of disability and death.
Three of the top ten leading causes of reduced life expectancy around the world are respiratory diseases, including chronic obstructive pulmonary disease (third), lower respiratory infections (fourth), and lung cancer (sixth).1 The COVID-19 pandemic exacerbated this by causing widespread disease and mortality.
Despite these statistics, the number of new pulmonary therapeutics reaching the market is only 3 %—for other disease therapeutics it is 6-14 %. 2 This very low attrition rate is due to the poor preclinical models available. Models span from expensive non-human in vivo experimentation to simplistic in vitro models. Neither can recapitulate the intricacies and complexities of human lung biology and immunology.
There is therefore a definitive and urgent need for new preclinical models capable of accurately mimicking the human lung and predicting reactions to novel therapeutics. This article outlines the development of two Innovative UK grant-funded lung-on-a-chip, or microphysiological system (MPS) models, to resolve this problem.
To map the distinct areas and physiology of the human lung, a bronchial and an alveolar airway model were built utilizing the PhysioMimix® Single-organ System and Multi-chip Barrier plates. These models were compared to traditional air-liquid interface (ALI) cultures in static 24-well conditions.
Further complexity was incorporated into the lung MPS models by including primary human pulmonary microvascular endothelial cells on the basolateral side of a perfused Transwell®. Also incorporated were monocytes, either basolaterally in the bronchial model to act as circulating immune cells, or on the apical side of the alveolar model to represent an alveolar macrophage-like cell.
The perfused MPS models mimicked human lung tissue accurately, showcasing relevant tissue architectures and cellular differentiation. The use of these models for respiratory infection research and development was validated with pseudotyped lentivirus expressing the SARS-CoV-2 Spike protein, which was used to infect the models, replicating the COVID-19 infection. The infection and resulting inflammatory responses were mapped, highlighting the relevance of the model when compared to patient inflammatory profiles in COVID-19 disease.
Neutralizing monoclonal antibody therapy, against the SARS-CoV-2 Spike receptor binding domain (RBD), was used to inhibit the infection. The model responded in a dose-dependent manner. This data demonstrates the model's ability to predict the efficacy of therapeutics against SARS-CoV-2. It also provided a better understanding of COVID-19 disease progression.
Lung MPS

Figure 1. A visual representation of the alveolar and bronchial MPS models. Using CN Bio's PhysioMimix® Single-organ System, alveolar and bronchial MPS models were made in Barrier plates. In the alveolar model, epithelial cells and THP-1 monocytes (alveolar macrophages) were cocultured on the apical side of the insert. In the bronchial model, epithelial cells were cultured on the apical side alone. In both models, lung endothelial cells were cultured on the basolateral side of the insert, and THP-1 monocytes were circulated with the perfused media. Image Credit: CN Bio
Results


Figure 2. Alveolar and bronchial cells cultured in MPS display superior tissue formation and differentiate into physiologically relevant cell phenotypes. (A) Alveolar tissues were sectioned and visualized using H&E staining. (B) qPCR analysis of alveolar cultures expression of AQP5 (AT1 cells) or SFTPB (ATII cells). (C) qPCR analysis of bronchial cultures expression of MUC5AC (goblet cells) or SCGB1A1 (club cells). (D) Bronchial tissues were sectioned and visualized using H&E staining. Scale bar, 50 μm. Image Credit: CN Bio


Figure 3. The addition of endothelial cells and monocytes allows the mapping of disparate inflammatory responses. (A) Confocal images of the cell layers in the alveolar model, with epithelial and monocyte (Mθ) on the apical side (top) and endothelial cells on the basal side (bottom). Monocytes were stained with CellTracker™ Far Red (orange) and all cells were stained with phalloidin (magenta) and DAPi (blue). Scale bar, 50 μm. (B) Bronchial cocultures with or without monocytes (Mθ) were challenged with LPS or poly (I:C) and IP-10 expression analyzed using ELISA over 48 hrs. (C) Alveolar cocultures with or without monocytes (Mθ) were challenged with LPS or poly (I:C) and IP-10 expression analyzed using ELISA over 48 hrs. Image Credit: CN Bio


Figure 4. Lung MPS are infected by SARS-CoV-2 and produce an inflammatory response. (A) ACE2 and TMPRSS2 mRNA expression in alveolar and bronchial MPS cultures was measured using qPCR. (B) Pseudotyped lentivirus expressing the SARS-CoV-2 Spike (D614G) protein tagged with mCherry was used to infect alveolar cultures. Tissues were fixed and stained for SFTPB (green), DNA (blue), and mCherry (red). Scale bar, 100 μm. (C) Pseudotyped lentivirus expressing the SARS-CoV-2 Spike (D614G) protein tagged with mCherry was used to infect alveolar cultures with THP-1 monocytes (tagged with far-red Cell Tracker (orange)). Tissues were fixed and stained for mCherry (red), actin (green), and DNA (blue). Scale bar, 100 μm. (D) TNFα, IL-1β, and IL-6 expression were measured using ELISA over 48 hrs during infection. Image Credit: CN Bio


Figure 5. Lung MPS predict the efficacy of COVID-19-neutralising antibodies. (A) Alveolar cultures were incubated with increasing concentrations of monoclonal antibody against the Spike RBD (0.05, 0.5, 5 μg/ml), before being infected using pseudotyped lentivirus expressing SARS-CoV-2 Spike (D614G) protein. Cultures were incubated for 48 hr before being fixed and stained for actin (green), DNA (blue), and mCherry (red). Scale bar, 100 μm. (B) Number of infection foci per image (10 images/condition). Image Credit: CN Bio
Conclusion
Innovate UK grant-funded the development of two lung-on-a-chip models designed to replicate the bronchial and alveoli airways for utilization in respiratory infection research and drug development. Compared to traditionally cultured cells at ALI, the perfused models showed greater tissue formation and differentiation into key cell types found in each region of the human lung.
The COVID-19 pandemic was a global wake-up call that highlighted the risks of new and evolving pathogens. To address this, and any future threats, new methodologies capable of accurately modeling and predicting diseases must be used.
Lung MPS are an answer to this demand. The two lung MPS model the human lung and exhibit human-relevant inflammatory responses to infection via pathogens. The evaluation of tissue and cell culture media can help us understand the biological mechanisms that cause disease and assist in predicting human reactions to medications to fight disease.
Together, the lung MPSs offer a solution to the need for faster identification and validation of clinical drug candidates, which enables us to confront emerging diseases with absolute precision.
References and further reading
- WHO Global Health Estimates, 2020
- Barnes et al., 2015
About CN Bio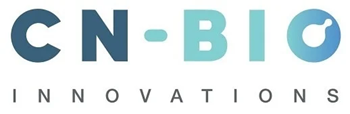
CN Bio is a leading organ-on-a-chip (OOC) company that offers a portfolio of products and contract research services to optimise the accuracy and efficiency of bringing new medicines to market. With more than a decade of research and development experience, we aim to transform the way human-relevant pre-clinical data is generated through the development of advanced in vitro human organ models.
CN Bio's PhysioMimix® OOC range of microphysiological systems (MPS) enable researchers to recreate human biology in the lab. The technology bridges the gap between traditional cell culture and human studies, to support the development of safer and more efficacious therapeutics, whilst reducing the dependence on animal model usage.
CN Bio’s portfolio of products (MPS, 3D validated cells, consumable plates) and services support researchers that require reliable, data-rich, in vitro studies, to uncover novel mechanistic insights into drug or disease mechanism of action.
Sponsored Content Policy: News-Medical.net publishes articles and related content that may be derived from sources where we have existing commercial relationships, provided such content adds value to the core editorial ethos of News-Medical.Net which is to educate and inform site visitors interested in medical research, science, medical devices and treatments.