The human lung is the only internal organ directly afflicted by the environment. It therefore faces a relentless war against foreign matter. For hundreds of years, lungs have been assaulted by both synthetic and natural enemies.
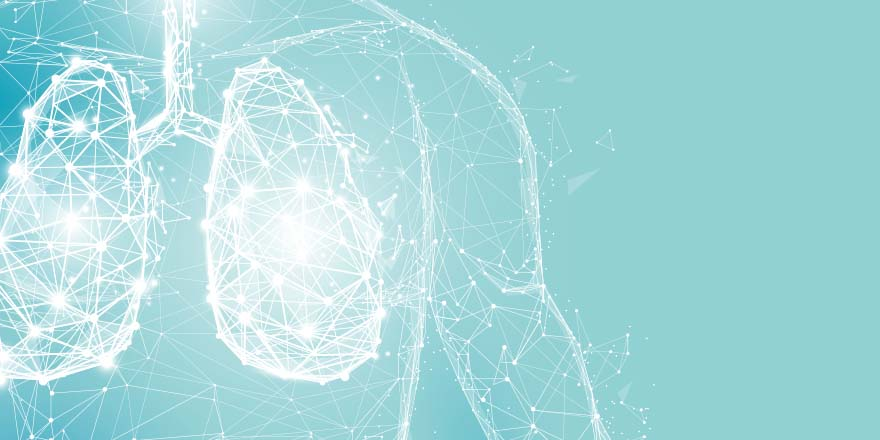
Image Credit: CN Bio Innovations Limited
Biological assailants like fungi, bacteria, and viruses continuously test natural defenses that have evolved over millennia. Newer and stranger particles that originate from burning fuels, harmful chemicals, and disintegrating plastics are also entering the lungs on a more frequent basis.
Consequently, lung diseases are now one of the largest causes of death; with lung cancer, chronic obstructive pulmonary disease (COPD), and lower respiratory infections ranking as three of the ten most common causes of death globally.1
The recent COVID-19 pandemic has exacerbated this global crisis. As of March 2022, the World Health Organization (WHO) reported approximately 450 million confirmed cases of COVID-19 and 6 million deaths.2
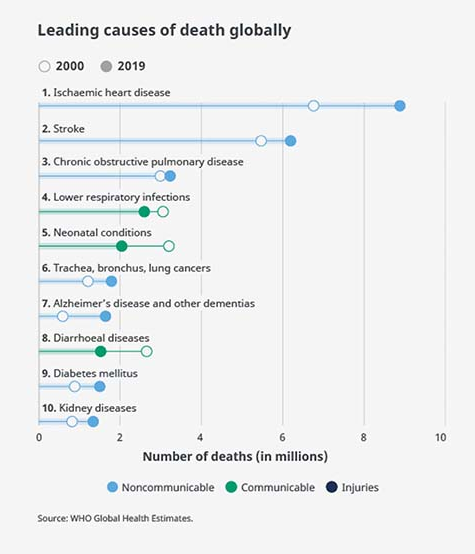
Figure 1. Leading causes of death globally. Source: WHO Global Health Estimates.
Thankfully, the lung is resilient and well-defended from encountered dangers. Goblet cells are specialized cells that produce a gel-like mucus layer that coats the airway epithelium. When large particulate matter enters the airway, it becomes entrapped in the mucus.
By the “beat” of the cilia (a brush-like structure formed by approximately 50 % of airway cells) the mucus is relocated back up the airway into the digestive system for destruction. This mucus cycle, or mucociliary clearance, stops most attacks to the lung—but not all.
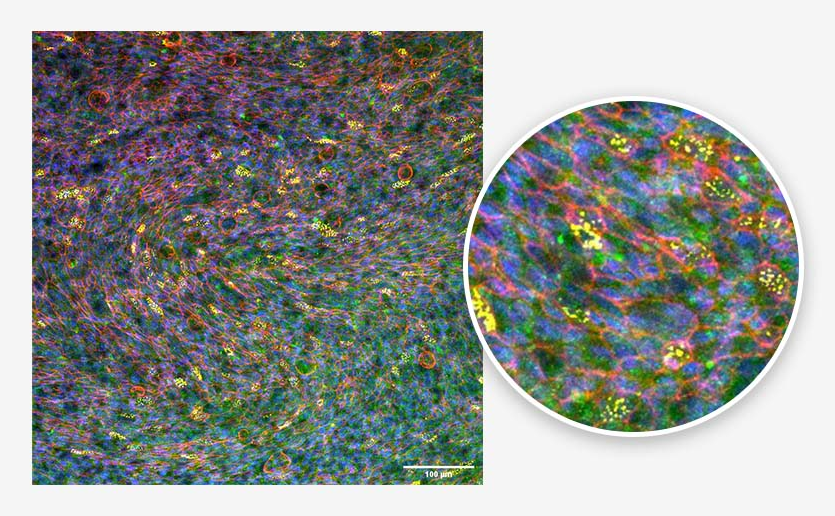
Figure 2. Bronchial epithelium. Tissue generated using the CN Bio PhysioMimix® OOC and Barrier (MPS-T12) Plates. Ciliated cells (yellow), mucus (green), actin cytoskeleton (red), and nuclei (blue) are indicated. Image Credit: CN Bio
Foreign bodies that evade frontline defences and reach the alveoli are only 0.2 μm away from meeting the bloodstream. However, alveolar macrophages (the first immune sentinel of the lung) prevent them from entering. Alveolar macrophages monitor the internal section of the alveoli and control the switch from anti-inflammatory tolerance to inflammatory attack response.
More severe inflammatory responses cause the infiltration of circulating immune cells from the systemic blood. These cells destroy the invader; however, this often results in damage to the alveoli as well.
The incorrect pathogen, coupled with inflammatory signaling from alveolar macrophages, could represent the difference between an average day and a journey to the emergency room.
This functional diversity, managed by a labyrinth of pulmonary and immune cells, has been difficult for scientists to recreate in the laboratory. Traditional in vitro models vary from basic 2D cell line cultures to 3D spheroids and scaffold models. While substantial efforts and great improvements have been made over time, standard approaches are unable to replicate many of the lung’s complex interactions.
To overcome these limitations, animal models are commonly utilized for lung research, despite differences in biology and physiology compared to the human lung.3 These differences manifest in relatively high drug attrition rates, with only 3 % of pulmonary therapeutics making it to market as compared to 6-14 % of therapeutics for other diseases.4
Ex vivo studies, which use isolated, perfused lungs or tissue slices from human sources, offer an option to limit animal experimentation and bring more physiological relevance to studies.5,6 However, entire human lung tissues can be hard to obtain, with precision-cut lung slices (PCLS) providing a more cost-effective alternative.
Table 1 summarizes the pros and cons of traditional approaches. The lack of readily accessible, cost-effective, human-representative preclinical models has caused preclinical pipeline bottlenecks and appalling drug attrition rates.
Table 1. The pros and cons of traditional preclinical lung models. Source: CN Bio
Model |
Advantages |
Disadvantages |
2D insert cell culture |
- Low cost
- High throughput
- Air-liquid interface (ALI) capacity
- Ability to coculture cell types
|
- Often cell lines–are not as representative as primary human cells
- Lack of 3D structure formation
- Long timeframe differentiation protocols
|
Spheroid/organoid culture |
- 3D integrated tissue formed
- Multiple cell types
- Use of stem cells / primary human cells
- ALI capacity
|
- Expensive and long timeframe experiments (stem cells)
- A bulk sphere of cells is not physiologically relevant to lung
|
Scaffold cultures |
- Scaffolds allow the formation of lung-relevant 3D architecture
- Cell-ECM interaction
|
- Currently challenging to monitor cells in scaffolds
- Post-culture analysis is difficult
|
Ex vivo |
- Full organ with heterogeneous cell population
- Human ex vivo lung is fully representative
- Lungs can be perfused post-isolation
- Precision-cut lung slices are available
|
- Difficult to obtain human tissue
- Small animal lung is not representative of human lung physiology
- Operates in isolation from the full system
|
In vivo |
- Ability to understand system biology interactions
- Full working organs with heterogeneous cell population
- Non-human primates have similar physiology to human lung
|
- Ethically questionable
- Expensive and time-consuming
- Low availability of non-human primates
- Small animal lung physiology is different from human
- Controlling studies at the cellular level and investigating molecular mechanisms and/ or genetic drivers is difficult
|
The air that we breathe has become pollutant-laden from modern conveniences, like cleaning fluids and solvents, vehicles, gas central heating, paints, and even farms. There is also a heightened risk of new pathogens causing future global pandemics. Quicker production of vaccines and therapeutics is therefore required.
Following the COVID-19 pandemic, this requirement is more understood by organizations. For instance, the Bill Gates Foundation and Wellcome Trust donated $300 million to the Coalition for Epidemic Preparedness Innovations (CEPI) to shorten the time needed to develop new vaccines.
The same level of preparation should be applied to the preclinical pipeline, whereby the effect of new irritants and pathogens can be quickly comprehended, and therapeutics can be designed and tested to predict their safety and efficacy in humans before final release to clinical trials.
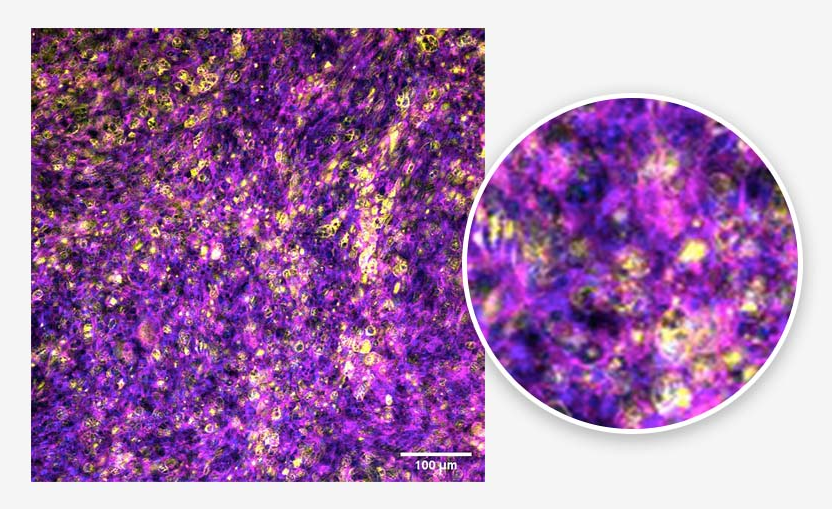
Figure 3. Alveolar epithelium. Tissue generated using the CN Bio PhysioMimix® OOC and Barrier (MPS-T12) Plates. ATII cells (yellow), actin cytoskeleton (magenta), and nuclei (blue) are indicated. Image Credit: CN Bio
To achieve this, cost-effective, human-relevant models that accurately recapitulate both diseased and healthy lung biology are urgently needed. Lung-on-a-chip models (LOAC), also known as lung microphysiological systems (MPS), have been in development over the last ten years. They have been shown to mimic aspects of the human lung better than others in vitro and could therefore offer a solution.
A large variety of LOAC models are now available, varying from single-use microscale chips to insert-based multi-well plate systems that offer a simpler way to incorporate immune cells and apply physiologically relevant infection parameters.7-10
LOAC models house a combination of integrated microfluidics, stretchable membranes, and scaffolds that expose cells to the shear stresses of the human lung, promoting the formation of microtissues that mimic the human lung’s pathophysiology, as well as integrated sensors to monitor the tissues in real-time.
The more flexible solutions are adaptable to increase model complexity, via the co-culture of a large array of cells, to address more specific research questions.11-13
Table 2. The pros and cons of next-generation LOAC. Source: CN Bio
Model |
Advantages |
Disadvantage |
Organ-on-a-chip (OOC) or microphysiological systems (MPS) |
- 3D integrated tissues form
- Use of human primary/stem cells with the capability to coculture multiple cell types
- Use of scaffolds
- ALI capability
- Perfused media or air provides shear stress for further differentiation potential
- Flexible membranes mimic the movement of the lungs during breathing
- Integrated sensors for impedance, barrier integrity, pH, microscopy
|
- Lower throughput and more expensive than standard cell culture
- Adoption barriers exist for OOC systems featuring non-standard cell culture designs
- Closed OOC systems are more difficult to infect and monitor inflammatory responses than open systems
- Some OOC solutions do not offer recirculating media/cannot incorporate immune cells into the model
|
With the capacity to recreate physiologically relevant human lung tissue and monitor tissues in real time, LOAC models work well in studies that investigate inflammatory and infection responses to foreign matter.14-16 Open-well format systems are valuable as test matter that can be delivered into the system as aerosols or small volumes at the ALI, and samples for analysis can be removed simply over time for longitudinal studies.
The potential safety and efficacy of developmental therapies can be tested via addition to the system apically at the ALI to mimic the dosing of inhaled medications, or basolaterally to simulate intravenous or oral therapies. Systemic effects can be elucidated by interconnecting relevant MPS organs together to create multi-organ systems.17,18 Table 2 offers a summary of the pros, cons, and considerations when choosing an OOC solution.
LOAC models offer a lot of promise, are they able to save the lungs from crisis? Do they represent the missing piece of the preclinical puzzle? Can the complimentary use of LOAC models alongside traditional approaches eliminate the preclinical bottlenecks? Further research and time will tell, but the future looks promising!
References and further reading
- WHO. (2020). WHO Global Health Estimates. https://www.who.int/news-room/fact-sheets/detail/the-top-10-causes-of-death
- WHO. (2022). WHO Coronavirus (COVID-19) Dashboard. https://covid19.who.int/
- Muñoz-Fontela, C., Dowling, W. E., Funnell, S. G. P., Gsell, P. S., Riveros-Balta, A. X., Albrecht, R. A., Andersen, H., Baric, R. S., Carroll, M. W., Cavaleri, M., Qin, C., Crozier, I., Dallmeier, K., de Waal, L., de Wit, E., Delang, L., Dohm, E., Duprex, W. P., Falzarano, D., … Barouch, D. H. (2020). Animal models for COVID-19. Nature 2020 586:7830, 586(7830), 509–515. https://doi.org/10.1038/s41586-020-2787-6
- Barnes, P. J., Bonini, S., Seeger, W., Belvisi, M. G., Ward, B., & Holmes, A. (2015). Barriers to new drug development in respiratory disease. In European Respiratory Journal (Vol. 45, Issue 5, pp. 1197–1207). European Respiratory Society. https://doi.org/10.1183/09031936.00007915
- Hocke, A. C., Suttorp, N., & Hippenstiel, S. (2017). Human lung ex vivo infection models. Cell and Tissue Research, 367(3), 511. https://doi.org/10.1007/S00441-016-2546-Z
- Viana, F., O’Kane, C. M., & Schroeder, G. N. (2021). Precision-cut lung slices: A powerful ex vivo model to investigate respiratory infectious diseases. Molecular Microbiology. https://doi.org/10.1111/MMI.14817
- Artzy-Schnirman, A., Zidan, H., Elias-Kirma, S., Ben-Porat, L., Tenenbaum-Katan, J., Carius, P., Fishler, R., Schneider-Daum, N., Lehr, C. M., & Sznitman, J. (2019). Capturing the onset of Bacterial Pulmonary Infection in Acini-on-Chips. Advanced Biosystems, 3(9), e1900026. https://doi.org/10.1002/ADBI.201900026
- Huh, D., Matthews, B. D., Mammoto, A., Montoya-Zavala, M., Yuan Hsin, H., & Ingber, D. E. (2010). Reconstituting organ-level lung functions on a chip. Science (New York, N.Y.), 328(5986), 1662–1668. https://doi.org/10.1126/SCIENCE.1188302
- Stucki, J. D., Hobi, N., Galimov, A., Stucki, A. O., Schneider-Daum, N., Lehr, C. M., Huwer, H., Frick, M., Funke-Chambour, M., Geiser, T., & Guenat, O. T. (2018). Medium throughput breathing human primary cell alveolus-on-chip model. Scientific Reports, 8(1). https://doi.org/10.1038/S41598-018-32523-X
- Zamprogno, P., Wüthrich, S., Achenbach, S., Thoma, G., Stucki, J. D., Hobi, N., Schneider-Daum, N., Lehr, C. M., Huwer, H., Geiser, T., Schmid, R. A., & Guenat, O. T. (2021). Second-generation lung-on-a-chip with an array of stretchable alveoli made with a biological membrane. Communications Biology, 4(1). https://doi.org/10.1038/s42003-021-01695-0
- Barkal, L. J., Procknow, C. L., Álvarez-Garciá, Y. R., Niu, M., Jiménez-Torres, J. A., Brockman-Schneider, R. A., Gern, J. E., Denlinger, L. C., Theberge, A. B., Keller, N. P., Berthier, E., & Beebe, D. J. (2017). Microbial volatile communication in human organotypic lung models. Nature Communications 2017 8:1, 8(1), 1–10. https://doi.org/10.1038/s41467-017-01985-4
- Humayun, M., Chow, C. W., & Young, E. W. K. (2018). Microfluidic lung airway-on-a-chip with arrayable suspended gels for studying epithelial and smooth muscle cell interactions. Lab on a Chip, 18(9), 1298–1309. https://doi.org/10.1039/C7LC01357D
- Sellgren, K. L., Butala, E. J., Gilmour, B. P., Randell, S. H., & Grego, S. (2014). A biomimetic multicellular model of the airways using primary human cells. Lab on a Chip, 14(17), 3349–3358. https://doi.org/10.1039/C4LC00552J
- Benam, K. H., Novak, R., Ferrante, T. C., Choe, Y., & Ingber, D. E. (2020). Biomimetic smoking robot for in vitro inhalation exposure compatible with microfluidic organ chips. Nature Protocols, 15(2), 183–206. https://doi.org/10.1038/S41596-019-0230-Y
- Deinhardt-Emmer, S., Rennert, K., Schicke, E., Cseresnyés, Z., Windolph, M., Nietzsche, S., Heller, R., Siwczak, F., Haupt, K. F., Carlstedt, S., Schacke, M., Figge, M. T., Ehrhardt, C., Löffler, B., & Mosig, A. S. (2020). Co-infection with Staphylococcus aureus after primary influenza virus infection leads to damage of the endothelium in a human alveolus-on-a-chip model. Biofabrication, 12(2). https://doi.org/10.1088/1758-5090/AB7073
- Zhang, F., Liu, W., Zhou, S., Jiang, L., Wang, K., Wei, Y., Liu, A., Wei, W., & Liu, S. (2020). Investigation of Environmental Pollutant-Induced Lung Inflammation and Injury in a 3D Coculture-Based Microfluidic Pulmonary Alveolus System. Analytical Chemistry, 92(10), 7200–7208.
- Chen, W. L. K., Edington, C., Suter, E., Yu, J., Velazquez, J. J., Velazquez, J. G., Shockley, M., Large, E. M., Venkataramanan, R., Hughes, D. J., Stokes, C. L., Trumper, D. L., Carrier, R. L., Cirit, M., Griffith, L. G., & Lauffenburger, D. A. (2017). Integrated gut/liver microphysiological systems elucidates inflammatory inter-tissue crosstalk. Biotechnology and Bioengineering, 114(11), 2648–2659. https://doi.org/10.1002/BIT.26370
- Edington, C. D., Chen, W. L. K., Geishecker, E., Kassis, T., Soenksen, L. R., Bhushan, B. M., Freake, D., Kirschner, J., Maass, C., Tsamandouras, N., Valdez, J., Cook, C. D., Parent, T., Snyder, S., Yu, J., Suter, E., Shockley, M., Velazquez, J., Velazquez, J. J., … Griffith, L. G. (2018). Interconnected Microphysiological Systems for Quantitative Biology and Pharmacology Studies. Scientific Reports 2018 8:1, 8(1), 1–18. https://doi.org/10.1038/s41598-018-22749-0
About CN Bio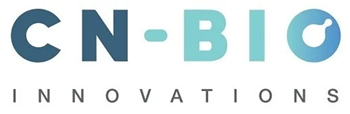
CN Bio develops human organ-on-chip technologies: devices that enable the formation of miniature models of human organs in the lab. We provide products and services to the pharmaceutical industry and in the past 3 years have used our proprietary organ-on-chip models in drug discovery and drug safety programs with more than 25 pharmaceutical companies. CN Bio has also pursued research to develop disease organ-on-chip models with successful programmes resulting in novel models of non-alcoholic steatohepatitis and Hepatitis B virus infection.
Working closely with academic pioneers in the bio-engineering field, and pharmaceutical and industrial partners, CN Bio continues to advance next generation human Organs-on-Chips.
Sponsored Content Policy: News-Medical.net publishes articles and related content that may be derived from sources where we have existing commercial relationships, provided such content adds value to the core editorial ethos of News-Medical.Net which is to educate and inform site visitors interested in medical research, science, medical devices and treatments.