Challenge
Non-invasive imaging of a patient-derived orthotopic mouse model of pancreatic cancer expressing GFP
Solution
In vivo imaging utilizing the UVP iBox Studio
Introduction
Since ancient times, the inherent glow of organisms has garnered the interest of observers1. In Chinese texts, references made in passing to glowing organisms can be found, for example in The Odes of Pin from 1500 to 1000 BCE‡,1,2: “Our paddocks seem crowded with deer, / glowing intermittently are the fireflies. / Such thoughts while they filled us with fear, / we tried, but in vain, to keep out.”
Almost 3000 years would pass until researchers found the source of the sporadic glow. Raphael Dubois, a French pharmacologist, created heat-sensitive and heat-labile extracts from an elaterid beetle, or firefly, of the Pyrophorous genus in 1885. He named the extracts luciferin and luciferase, respectively.
The two extracts generated a blue glow when combined. Over the next century, researchers found comparable examples of this chemical luminescence in different organisms. Luciferin oxidized to emit blue light in the presence of oxygen and luciferase.3 (Figure 1).
Still, the origin of a particular type of bioluminescence was unknown to researchers for almost another century.
While the majority of luminescent extracts emulated the hue that was seen in vivo, some did not. Organisms like jellyfish, or Aequorea Victoria, produced a green glow. It was possible to maneuver this glow by electrical or mechanical stimulation and it was visibly green beneath an ultraviolet (UV) mineralite4.
A scientific triumph was achieved in 1962 when Shimomura and his colleagues extracted the green-glowing organs of more than 10,000 jellyfish, which yielded just 5 mg of partially purified protein5. These extracts, known as ‘squeezates’, luminesced blue similar to the other bioluminescent extracts.
Shimomura established that because these extracts did not react with luciferin, they were not enzymatic. In contrast to the luciferin-luciferase pair (Figure 1A), the reaction did not require oxygen and only needed calcium. In doing so, he found the most basic luminescent system so far (Figure 1B).
Crucially, his blue luminescent extract, which he named aequorin, would emit a green glow when subjected to UV light. He continued to give a prophetic concluding statement regarding the green copurified protein in his 1962 paper5: “…it is reasonable to suppose that the greenish quality results from a light-filtering effect and fluorescence of the green protein which is highly concentrated together with aequorin in the photogenic cells. If this supposition is correct, perhaps the green protein has some biological significance, through its influence on the quality of the light of bioluminescence.”
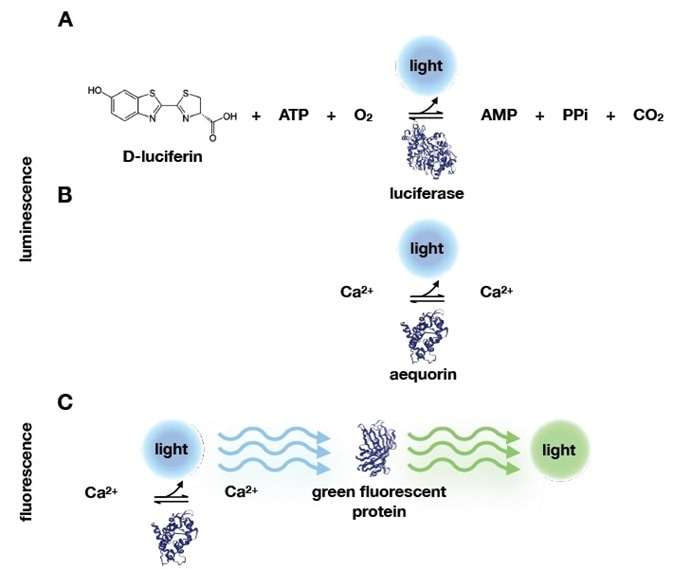
Figure 1. Bioluminescence and fluorescence reactions. A) Before Shimomura’s work, bioluminescence reactions were known to require ATP, O2 and the D-luciferin substrate. D-luciferin is oxidized during the enzymatic reaction with luciferase, which produces light. Shimomura and colleagues identified a simpler system, that only required calcium and aequorin. B) In addition, they discovered that the blue light emitted from calcium binding to aequorin could be absorbed by another protein that would emit green light. This protein was therefore called green fluorescent protein. Protein structures‡‡ in this image were retrieved from the protein data bank (PDB): luciferase (2D1R), aequorin (1EJ3), and green fluorescent protein (1EMB). Image Credit: Analytik Jena US
Shimomura would go on to show that when excited with UV, aequorin would contain an emission spectrum that overlapped with the green fluorescent protein’s (GFP) absorption spectrum. He also found that an intermolecular energy transfer between the two proteins was the cause of the green fluorescence6 (Figure 1C).
For his contributions, Shimomura was one of three scientists who were awarded the 2008 Nobel Prize in Chemistry7.
The present-day in vivo imaging community has been a key benefactor from the discovery of GFP, the characterization and cloning of the protein8, along with the extension of the fluorescent protein color pallete9,10. These fluorescent proteins bring light to darkness and make the invisible visible.
Presented below is the exceptional detail of the UVP iBox Studio when carrying out in vivo imaging of an orthotopic mouse model of pancreatic cancer labeled with GFP.
Orthotopic mouse model
See Lwin et al (2018) for a thorough guideline on animal care and use11. Mice were anesthetized with a combination of xylazine (10mg/kg), acepromazine (3mg/kg), and ketamine (100mg/kg). They were then laid on top of an Analytik Jena warming plate that had been pre-calibrated (part no. 95-0538-01).
A GFP emission filter (part no. 38-0352-01) was installed in the filter wheel and GFP was excited with blue epi-LED illumination. The image was taken with the following configurations: histogram – Auto, focus 74%, brightness/aperture 61%, binning – 1x1 and exposure time – 2.1 s.
A white light image was taken with the following configurations: histogram – Auto, focus 74%, brightness/aperture 20%, binning – 1x1, and exposure time – 70 ms.
The GFP fluorescent image was pseudocolored green. The fluorescent image and white light were combined to produce an image overlay. The acquisition of the image and post-processing was carried out utilizing the VisionWorks software package version 9.0 from Analytik Jena.
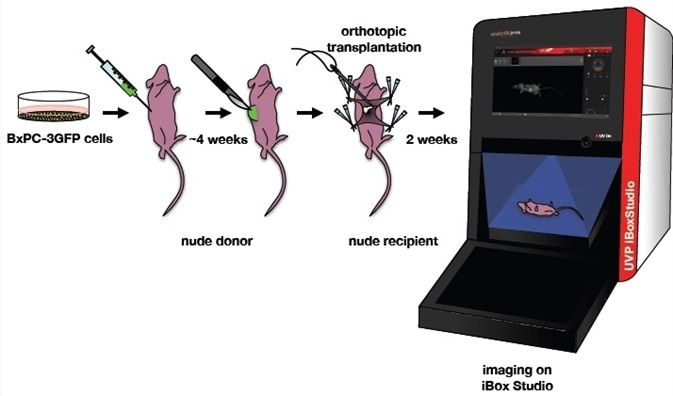
Figure 2. Orthotopic mouse model of pancreatic cancer. BxPC-3GFP human pancreatic cancer cell lines were maintained under selection. A nude donor mouse was injected with these cells. A piece of the GFP tumor was excised from the donor and implanted onto the tail of the pancreas in a nude recipient. Two weeks after implantation, the mouse was imaged on a UVP iBox Studio. Image Credit: Analytik Jena US
Results and conclusion
Two weeks after the orthotopic transplantation, non-invasive imaging was performed, which showed that the GFP signal continued to be significant even though it had traveled through multiple tissue layers (Figure 3).
This investigation demonstrates the precise detail that can be acquired with Analytik Jena’s deeply cooled, high sensitivity, low noise detector, along with the exceptional detection capability of the instrument to visualize a deep tumor.
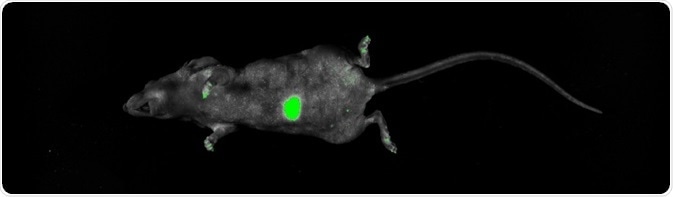
Figure 3. Imaging of GFP orthotopic mouse model. Imaging was performed on the UVP iBox Studio. The mouse was anesthetized according to Lwin et al (2018). The mouse was placed on top of warming plate during capture, GFP was excited using standard blue overhead LED illumination and a green (GFP) emission filter. The image was post-processed and composited using Analytik Jena’s VisionWorks software package ver. 9.0. Image Credit: Analytik Jena US
The UVP iBox Studio is able to benefit any in vivo imaging application. The instrument has been outfitted with red, green, blue, and white epi-LED illumination. An external xenon-arc lamp can be introduced for customized applications that demand stronger lighting.
Analytik Jena has created the UVP iBox Studio near-IR ready as researchers are increasingly adopting deep tissue non-invasive imaging. To excite 700 or 800 nm dyes, researchers can upgrade to near-IR lasers.
For researchers utilizing gaseous anesthesia, the UVP iBox Studio is supplied with gas ports, to allow for an external anesthesia system, which is offered by Analytik Jena as an add-on feature.
The filter wheel is also simple to access to ensure that users can outfit their UVP iBox Studio with emission filters from the expansive library from Analytik Jena or can purchase customized filters for specific applications whenever required.
The instrument’s customizability along with its simple to use interface and sensitivity makes it an ideal choice for researchers requiring a solution for high quality in vivo imaging.
References and Further Reading
- Harvey, E. N. A history of luminescence from the earliest times until 1900. 44, (American Philosophical Society, 1957).
- The Book of Poetry: Book XV. The Odes of Pin: III. Tung Shan. Available at: https://www.sacred-texts.com/index.htm. (Accessed: 1st October 2019)
- Dubois, R. Fonction photogenique des Pyrophores. CR Seances Soc Biol Fil 37, 559–62 (1885).
- Davenport, D. & Nicol, J. C. Luminescence in hydromedusae. Proceedings of the Royal Society of London. Series B-Biological Sciences 144, 399–411 (1955).
- Shimomura, O., Johnson, F. H. & Saiga, Y. Extraction, Purification and Properties of Aequorin, a Bioluminescent Protein from the Luminous Hydromedusan, Aequorea. Journal of Cellular and Comparative Physiology 59, 223–239 (1962).
- Morise, H., Shimomura, O., Johnson, F. H. & Winant, J. Intermolecular energy transfer in the bioluminescent system of Aequorea. Biochemistry 13, 2656–2662 (1974).
- The Nobel Prize in Chemistry 2008. Available at: https://www.nobelprize.org/prizes/chemistry/2008/summary/. (Accessed: 30th September 2019)
- Chalfie, M., Tu, Y., Euskirchen, G., Ward, W. W. & Prasher, D. C. Green fluorescent protein as a marker for gene expression. Science 263, 802–805 (1994).
- Campbell, R. E. et al. A monomeric red fluorescent protein. PNAS 99, 7877–7882 (2002).
- Shaner, N. C. et al. Improved monomeric red, orange and yellow fluorescent proteins derived from Discosoma sp. red fluorescent protein. Nat Biotechnol 22, 1567–1572 (2004).
- Lwin, T. M. et al. Tumor-Specific Labeling of Pancreatic Cancer Using a Humanized Anti-CEA Antibody Conjugated to a Near-Infrared Fluorophore. Ann Surg Oncol 25, 1079–1085 (2018).
- Head, J. F., Inouye, S., Teranishi, K. & Shimomura, O. The crystal structure of the photoprotein aequorin at 2.3 A resolution. Nature 405, 372–376 (2000).
- Brejc, K. et al. Structural basis for dual excitation and photoisomerization of the Aequorea victoria green fluorescent protein. Proc.Natl.Acad.Sci.USA 94, 2306–2311 (1997).
- Nakatsu, T. et al. Structural basis for the spectral difference in luciferase bioluminescence. Nature 440, 372–376 (2006).
‡ As translated by John Legge in The Books of Poetry and reinterpreted by Dr. Hu Shih as written in E. Newton Harvey’s 1957 book, A History of Luminescence from the Earliest Times Until 1900.
‡‡ Protein structures were retrieved from the PDB and reprinted here per their usage policy.
About Analytik Jena US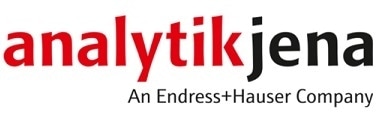
Analytik Jena is a provider of instruments and products in the areas of analytical measuring technology and life science. Its portfolio includes the most modern analytical technology and complete systems for bioanalytical applications in the life science area.
Comprehensive laboratory software management and information systems (LIMS), service offerings, as well as device-specific consumables and disposables, such as reagents or plastic articles, complete the Group’s extensive range of products.
About Life Science
The Life Science product area demonstrates the biotechnological competence of Analytik Jena AG. We provide a wide product spectrum for automated total, as well as individual solutions for molecular diagnostics. Our products are focused to offer you a quality and the reproducibility of your laboratory results. This will surely ease your daily work and speed up your work processes in a certain way.
All together we support you through the complete process of the lab work. Besides we offer customized solutions and are able to adapt our products to your needs. Automated high-throughput screening systems for the pharmaceutical sector are also part of this segment’s extensive portfolio.
About Analytical Instrumentation
Analytik Jena has a long tradition in developing high-performance precision analytical systems which dates back to the inventions made by Ernst Abbe and Carl Zeiss. We have grown to become one of the most innovative manufacturers of analytical measuring technology worldwide.
Our business unit Analytical Instrumentation offers excellent competencies in the fields of optical spectroscopy, sum parameters and elemental analysis. Being proud of our core competency we grant all our customers a long-term warranty of 10 years for our high-performance optics.
About Lab Automation
With more than 25 years of market experience, Analytik Jena with its CyBio® Product Line is a leading provider for high quality liquid handling and automation technologies. In the pharmaceutical and life science industries, our products enjoy the highest reputation for precision, reliability, robustness and simplicity.
Moreover, the Automation Team designs, produces and installs fully automated systems tailored to our clients' application, throughput and capacity requirements. From stand-alone CyBio® Well up to fully customized robotic systems we handle your compounds, biomolecules and cells with great care.
Sponsored Content Policy: News-Medical.net publishes articles and related content that may be derived from sources where we have existing commercial relationships, provided such content adds value to the core editorial ethos of News-Medical.Net which is to educate and inform site visitors interested in medical research, science, medical devices and treatments.