What is Gene Delivery?
A person’s genes define who they are and regulate the pathways which support life, such as movement, energy provision, and growth. The proteins they encode must obtain the precise structure that allows them to bind specifically with their intended target. A single mutation can stop a protein from executing its specific role, sometimes with devastating consequences.
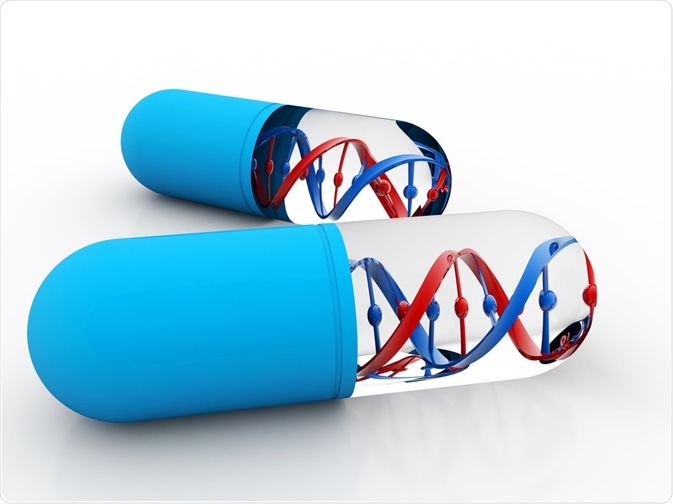
Image credits: Blackboard | Shutterstock
A number of disorders and diseases come from genetic mutations which hinder the production of a protein with a key function. The capability to replace or alter defective genes provides the potential to cure a wide scope of serious disorders, a number of which are otherwise incurable.
For a number of decades, such gene therapy has been a medical goal. Recent advances in genomic methods which allow precise manipulation of gene sequences have supplied renewed hope of ground-breaking genetic treatments becoming more readily available to enhance, and even save, the lives of patients suffering from genetic diseases.
A wide scope of gene therapies are being researched, but one of three key approaches is generally followed1. A mutated gene may be replaced by a healthy copy of the gene or inactivated to stop translation of the malfunctioning protein.
Copies of the required gene may be introduced into the body if a vital gene is not working or absent. Gene therapy is still an experimental method despite its huge therapeutic potential, and much research still needs to be carried out to ensure its long-term safety.
Gene therapy is currently limited to diseases for which there is no cure because of this. The indications for which gene therapy is currently being researched include HIV, sickle cell anaemia, haemophilia, cystic fibrosis, muscular dystrophy and some forms of cancer.1
Over 2,500 gene therapy clinical studies have been initiated over a wide range of indications, from infectious diseases to complex neurodegenerative disorders and cancer2.
A mutation of the gene that produces the cystic fibrosis transmembrane conductance regulator (Cftr) causes cystic fibrosis. Gene therapies include genome editing to permanently remove mutations in the Cftr gene and the introduction of healthy Cftr genes3.
In the same way, gene therapies have been developed to repair or replace malfunctional dystrophin genes in Duchenne muscular dystrophy4,5.
Anticancer gene therapies which augment drug effects, heighten the immune response, or interfere with tumor defence mechanisms have proved effective in the treatment of a number of types of cancer, these include melanoma6, prostate cancer7 and lymphoma8.
Research is ongoing at an unprecedented rate, developing better targeted and more effective ways to deliver gene therapy. There has been increasing focus on non-viral gene delivery vehicles, such as nanoparticles most recently9.
Non-viral gene delivery systems have shown great potential as an alternative strategy for tissue repair and regeneration, in addition to disease-modifying treatments10.
Methods of Gene Delivery
A key part of establishing the efficacy of gene therapy is how the gene is introduced. A vector is required to deliver the therapeutic gene (to restore a missing/malfunctional protein), or therapeutic RNA (used to modify transcription of an existing gene) into the nucleus, since genetic material introduced directly into a cell is usually not transcribed.
The vector can be injected in vivo, either directly or intravenously into a particular tissue, or mixed with a sample of the patient's cells which are later returned to the patient.
Generally, the former is utilized in order to target long-lived post-mitotic cells, whereas the ex vivo method is required to infect haematopoietic and other stem cells.
It is vital that the vector does not prompt an immune response and delivers its genetic cargo to the correct cell without interfering with the functioning of other genes in order for the gene therapy to be successful.
A number of the children who underwent gene therapy for severe combined immunodeficiency (SCID) subsequently developed T cell leukaemia because of insertional mutagenesis11. To produce the required protein an introduced gene must also be transcribed effectively.
As they possess a natural ability to deliver viable genetic material into cells, viruses were a clear choice of vector. The therapeutic gene is inserted into the viral DNA and the disease-causing genes inactivated. As the virus infects a cell, it delivers the inserted gene together with its own, but does not result in disease.
Adenoviruses are the viruses which are most frequently utilized as gene delivery vectors. They introduce their DNA into the nucleus of the infected cell, and retroviruses, which integrate their genetic material into the host chromosome.
The limitations of viral vectors powered the search for alternative non-viral gene delivery systems possessing better cell specificity, better modifiability, lower immunogenicity and toxicity, and higher productivity.
These include physical techniques, for example jet injection, biolistics, and ultrasound, that allow direct penetration into the cytosol. These physical systems had the advantage of reduced toxicity, but since much of the introduced DNA was degraded before it reached the nucleus, transfection efficacy was low12,13.
Using compounds such as calcium phosphates, lipids and cationic polymers, chemical transfection was also researched but was associated with toxicity14.
Recent successes in tailoring molecular size and structure have decreased endosomal degradation and toxicity, and heightened the efficiency of non-viral transfection, making them a good alternative to viral vectors10.
Nanoparticle Gene Delivery Systems
In recent years, the field of nanotechnology has been growing quickly, supplying novel compounds with unique properties to satisfy a large scope of applications. Due to their high surface area-to-volume ratio, and small size (1 to 100 nm), nanomaterials have good potential as effective non-viral gene delivery systems15,16.
Even though transfection efficacy is still lower than with viral vectors, they are not as susceptible to reticuloendothelial clearance and demonstrate better internalization and uptake than standard non-viral vectors.
In addition, nanoparticles may be designed to be responsive to an external stimulus or be combined with a specific ligand to allow the site and time of gene delivery to be precisely controlled17.
A number of materials (both inorganic and organic) have been assessed for nanoparticle gene delivery, including gold, lipids, graphene, peptides and other polymers.
The genetic material can either be encapsulated within the nanoparticle or conjugated to it via electrostatic binding between the negative charges of the DNA and the positive charges of the nanoparticle.
Nanoparticles of the amine polymer polyethylenimine have been examined extensively, and are most effective when conjugated to polyethylene glycol (PEG) to extend blood circulation time, decrease non-specific interactions with serum proteins, and facilitate accumulation in targeted tissues15.
PEGylated-polyethylenimine-cholesterol (PEG-PEI-cholesterol) gene delivery enhanced expression of the cytokine interleukin 12 successfully, in a clinical trial of epithelial ovarian immunotherapy18.
Due to their desirable biodegradability and biocompatibility properties, proteins have also been chosen as gene delivery vectors. Additionally, their amphilicity enables them to interact with both the solvent and the genetic cargo.
To optimize functionalization and incorporate additional desirable properties, peptides and proteins are frequently combined with inorganic nanomaterials. A system comprising protamine sulphate and gelatin has attained efficient gene delivery19.
Similarly, Tat peptide conjugated with silver nanoparticles allowed the delivery of DNA to the nucleus of stem cells with low cytotoxicity20. As it supplies protection from nucleases, high drug loading efficiency, and controlled release, the unique properties of the carbon allotrope graphene make it a particularly attractive gene carrier.
Infrared-stimulated gene delivery was acquired with a polyethylene glycol-branched polyethylenimine-reduced graphene oxide (PEG-BPEI-rGO)21. Despite the huge potential of nanoparticles as vectors for gene therapy, none have yet been licensed for clinical use.
This is mainly because of concerns regarding potential health risks that need further assessment, such as direct effects on the central nervous system and body distribution and deposition.
Characterization of Nanoparticles
Nanoparticles are characterized in terms of their agglomeration state, size, surface composition or shape. Some of these characteristics have an effect on their optical properties.
For that reason, UV/vis absorbance is utilized to establish the specific absorbance profile of a nanoparticle suspension and to ascertain their concentration. The analysis can be carried out in 96-well microplates using microplate readers equipped with a UV/vis spectrometer.
The technique is suitable for high throughput analysis of a nanoparticle suspension, as the absorbance spectrum of a single well is recorded in under a second. The technique was further used by researchers from Texas University: the microplate-based characterization method determined the yield of nanoparticle biosynthesis22.
A further study characterized the cargo of nanoparticles by coupling the cargo (siRNA) to a fluorophore. Measuring the fluorescence intensity of nanoparticles in a CLARIOstar microplate reader revealed the efficiency of non-covalent coupling of siRNA to gold nanoparticles23.
Liposome Gene Delivery Systems
Due to their biocompatibility, efficacy and targeting ability – liposomes, closed concentric vesicles of phospholipids, are well established as versatile drug carrier systems23.They are also able to transport DNA,making them potential non-viral vectors for gene delivery24,25.
Due to their capacity to accommodate much larger pieces of DNA compared with viral vectors, and the safety advantage – complexes of cationic phospholipids and a functional gene or DNA, known as genosomes, have been examined as gene delivery vectors.
During circulation, by bypassing the cell membrane, the lipids protect the DNA from degradation and promote its delivery. With enhanced liposome formulations, like the utilization of additives such as cholesterol to decrease leakage and hemagglutinating virus of Japan to improve transfection, they constitute a valuable alternative to viral vectors for gene therapy.
Often, PEG is added to liposomes in order to improve colloidal stability and reduce aggregation to extend their circulation half-life25. It is now known that such PEGylation of liposomes results in the spontaneous formation of lipid nanoparticles, which can decrease transfection efficacy.
By attaching targeting ligands to PEGylated complexes this can be restored, which has the added advantage of allowing targeted delivery and receptor-mediated uptake. Research continues to develop genosome formulations with less clearance by the reticuloendothelial system and better transfection rates25.
Genosomes made up of helper lipids of unsaturated dioleoyl chains and phosphatidylethanolamine head groups improved transfection efficiencies of DOPC/DOPE/SS14 lipoplexes significantly24.
In the same way, genosomes prepared with gemini surfactants (two or more head groups and two aliphatic chains linked by a spacer) attained greater transfection efficiency27,87.
Encapsulation oligonucleotides in stabilized antisense lipid particles has also been revealed to be associated with enhanced cellular uptake29. Most recently, the benefits of liposomes have been combined with those of nanomaterials with the creation of solid lipid nanoparticles (SLNs) comprising surfactant-stabilized lipids.
Studies have shown that SLNs supply effective long-acting and high-capacity non-viral gene delivery with better transfection efficacy31,30. These improvements in liposome structure have escalated their potential as gene delivery vectors.
Yet, their utilization in a clinical setting may only be realized after further research has explained the mechanisms of interaction between lipid vectors and gene expression in vivo, plus their safety profiles.
Microbubble Gene Delivery Systems
Physical techniques, for example ultrasound, can also be utilized to deliver DNA into a cell by heightening cell membrane permeability. Using microbubbles (gas-filled spherical voids less than 5 μm in diameter stabilized by a material coating composed of a phospholipid or synthetic polymer), the uptake of DNA by sonoporation has been increased.
The microbubbles collapse quickly at high-amplitude, low frequency ultrasound, generating liquid jets that can penetrate cells by prompting endocytotic uptake and creating transient pores in the cell membrane31.
Ultrasound itself can be locally targeted to the desired tissue, but the employment of microbubbles can facilitate more selective gene delivery through the incorporation of targeting ligands on their surface. The ligands may be peptides, antibodies, or vitamins, depending on the chosen target.
Maximum gene expression when employing microbubble-mediated gene delivery has been revealed to be over 700 times more than ultrasound alone33. The method transfected inhibitory RNA into tumor cells successfully, reducing tumor growth and preventing angiogenesis34 and achieved gene delivery to mouse myocardium354.
In addition, microbubbles have also been utilized to deliver genes to damaged liver to prevent or delay the development of liver fibrosis by regulating the expression of exogenous genes36.
This method has delivered viral DNA vectors to the brain parenchyma successfully, which is otherwise impossible because of the blood-brain barrier, opening up the possibility of gene therapy for genetic disorders of the central nervous system37.
The gene transfection efficiency and binding capacity of microbubbles has been increased further by heightening the cationic charge and this has exhibited increased therapeutic effect38.
Compared with neutral microbubbles, the expression of reporter gene delivered by cationic microbubbles was 20 times more in vitro and 3 times more in a tumor model 39. Microbubbles have been combined with nanotechnology to create nanobubbles, as with liposomes38.
These have the added benefit of being able to cross through the blood vessels and attain better tumor permeability and retention. Impressive transfection efficacy was seen when ultrasound combined with nanobubbles was employed to deliver the tumor necrosis factor to tumor-bearing mice.
Treatment led to lower tumor vessel density and inhibition of tumor growth40. Yet, it has also been reported that higher microbubble concentrations decreased cell viability significantly, without increasing transfection efficiency, and prolonged ultrasound exposure lowers the efficiency of gene transfection32.
In addition, ultrasound-mediated microbubble gene delivery is a multistep, complexprocess and more research is needed to understand each of the steps in the process in order to facilitate optimization of the method for clinical applications40.
Viral Gene Delivery Systems
Viral vectors have been based on a number of virus types, but the most frequently utilized are adenoviruses, retroviruses, adeno-associated viruses (AAV), and lentiviruses. Different viruses permit the delivery of different types of genetic material including both double- and single-stranded DNA or RNA41.
Viral vectors are still the most efficient way of delivering genetic material into host cells. Yet, the progress of other viral gene therapies was halted in favor of safer but less effective non-viral vectors because of cases of leukaemia and the death of a young patient after receiving gene therapy in the 1990s41.
The advances in genetic engineering which permit precision splicing and a greater understanding of gene structure and expression have recommenced research efforts into viral gene therapy.
A large amount of gene therapy clinical trials have now been performed with a range of non-pathogenic, replication-defective viral vectors over a number of indications41, and the results are promising.
For instance, intravenous administration of a tumor-selective chimeric adenovirus vector into 17 patients with cancer led to tumor-specific delivery with no treatment-related serious adverse effects42.
Stem cell-based lentiviral gene therapy has led to life-long production of Factor VIII, exhibiting the potential to cure haemophilia A43. Clinical trials are ongoing for gene therapy lentivirus vectors to inhibit CCR5 and give protection against HIV44.
There are now approved viral gene therapies which are being utilized successfully in clinical practice45. These include a cancer treatment which inserts the CD19 gene into the patient's T cells ex vivo; when reintroduced the T cells are able to more effectively destroy cancer cells.
For the treatment of blood cancers, donor lymphocytes transfected with Herpes simplex virus-1 thymidine kinase and truncated low affinity nerve growth factor receptor are being utilized in the same way.
A plasmid vector carrying the vascular endothelial growth factor (VEGF) gene, which is directly injected into the target ischaemic tissue, though not yet approved, is currently under development to cure muscular dystrophy and cystic fibrosis45.
Although there has been a lot of progress, more research is still needed to address concerns around current disadvantages of viral delivery, both perceived and real.
These concerns include immunogenicity, off-target delivery, carcinogenesis, random integration of some viral vectors into the host chromosome, and the limitations in the size of the nucleic acid that can be packaged and employed for viral gene therapy45.
So, the safety of viral vectors must be proven before they will again be fully trusted as the most competent gene delivery, even though they provide better transfection efficiency than non-viral vectors.
How a Microplate Reader Assists in Gene Delivery Research
Measuring 96 or more samples in a single run is made possible by microplate readers. This makes them ideal for examining different conditions in a singular experiment. This advantage is utilized in gene delivery research to study the effectiveness of a gene delivery technique at a cellular level.
For example, cancer targeting gene delivery uses cell viability assays like the fluorescent Resazurin assay or the colorimetric MTT assay to establish whether cancer cells die after exposure to the cancer-targeted gene delivery system47.
A microplate reader enables an even closer look at how gene delivery systems influence cells. For instance, themicroplate-based mitochondrial membrane potential (MMP) assay employing Rhodamine 12348 can be utilized to report on mitochondria integrity and cell metabolism in response to gene delivery systems.
Apart from cellular assays, microplate readers also establish the amount of cargo specific delivery systems can carry. Usually, this is performed by labeling the nucleic acid with a fluorophore and acquisition of the fluorescence intensity of the delivering particles.
Conclusion
Dysfunctional genes make up 80% of over 7,000 known diseases, and nearly 350 million people are affected by genetic diseases worldwide. Gene therapy supplies the way to correct such disease and has huge medical potential.
Gene therapy provides hope of a life-long cure, unlike current treatments for a lot of diseases which merely delay progression or manage the symptoms. Difficulties in achieving sustained expression of transgenes, paired with the lack of a system to provide the curative genetic material to the affected cells that is both efficient and safe, has hindered advancements in gene therapy.
Viral vectors supply the effective transfection of engineered DNA/RNA but are linked with concerns about safety and limitations to the amount of nucleotides which can be incorporated into the viral genome.
Even though there has been huge amounts of progress in the development of non-viral gene vectors as researchers work to realize more genetic therapies, low transfection rates still limit their progress to clinical employment.
Recent advances in genomic manipulation and the development of new formulations of non-viral transfection vectors have resulted in the approval of a number of gene therapies. There is real hope that additional research will result in the development of a large variety of life-changing gene therapies.
Via the provision of high-quality laboratory equipment, this research is being facilitated by BMG Labtech. Microplate readers allow the efficient assessment of genetic manipulations, to maximize research efforts.
References
- U.S. National Library of Medicine. How does gene therapy work? August 6 2019. https://ghr.nlm.nih.gov/primer/therapy/procedures
- Xavier M, et al. Entering the Modern Era of Gene Therapy. Annu. Rev. Med. 2019. 70:273–288. https://www.annualreviews.org/doi/pdf/10.1146/annurev-med-012017-043332
- Yan Z, et al. Advances In Gene Therapy For Cystic Fibrosis Lung Disease. Hum Mol Genet. 2019 Jul 23. Epub ahead of print]. https://www.ncbi.nlm.nih.gov/pubmed/31332440
- Alfano LN, et al. Long-term treatment with eteplirsen in nonambulatory patients with Duchenne muscular dystrophy. Medicine (Baltimore). 2019 Jun;98(26):e15858. https://www.ncbi.nlm.nih.gov/pubmed/31261494
- Meng J, et al. Autologous skeletal muscle derived cells expressing a novel functional dystrophin provide a potential therapy for Duchenne Muscular Dystrophy. Sci Rep. 2016; 6: 19750. https://www.ncbi.nlm.nih.gov/pmc/articles/PMC4728433/
- Perez MC, et al. Observational study of talimogenelaherparepvec use for melanoma in clinical practice in the United States (COSMUS-1). Melanoma Manag. 2019 Jul 3;6(2):MMT19. https://www.ncbi.nlm.nih.gov/pubmed/31406563
- Twardowski P, et al. Randomized phase II trial of sipuleucel-T immunotherapy preceded by sensitizing radiation therapy and sipuleucel-T alone in patients with metastatic castrate resistant prostate cancer. Cancer Treat Res Commun. 2019;19:100116. https://www.ncbi.nlm.nih.gov/pubmed/30682445
- Viardot A, et al. Chimeric antigen receptor (CAR) T-cell therapy as a treatment option for patients with B-cell lymphomas: perspectives on the therapeutic potential of Axicabtageneciloleucel.Cancer Manag Res. 2019 Mar 25;11:2393-2404. https://www.ncbi.nlm.nih.gov/pubmed/31114317
- Chang J-H, et al. Sleeping Beauty Transposon-Mediated Asparaginase Gene Delivery by a Nanoparticle Platform. Scientific Reports 2019;9:11457. https://www.nature.com/articles/s41598-019-47927-6
- Wu P, et al. Non-viral gene delivery systems for tissue repair and regeneration. Journal of Translational Medicine 2018;16:29. https://translational-medicine.biomedcentral.com/articles/10.1186/s12967-018-1402-1
- Hacein-Bey-Abina S, et al. Insertional oncogenesis in 4 patients after retrovirus-mediated gene therapy of SCID-X1. J. Clin. Investig. 2008;118:3132–3142. https://www.ncbi.nlm.nih.gov/pmc/articles/PMC4947087/
- Robinson HL, Pertmer TM. Nucleic acid immunizations. Curr Protoc Immunol. 2001 May;Chapter 2:Unit 2.14. https://www.ncbi.nlm.nih.gov/pubmed/18432766/
- Panje CM, et al. Ultrasound-mediated gene delivery with cationic versus neutral microbubbles: Effect of DNA and microbubble dose on in vivo transfection efficiency. Theranostics. 2012;2(11):1078–1091. https://www.ncbi.nlm.nih.gov/pmc/articles/PMC3516840/
- Luten J, et al. Biodegradable polymers as non-viral carriers for plasmid DNA delivery. J Control Release. 2008 Mar 3; 126(2):97-110. https://www.ncbi.nlm.nih.gov/pubmed/18201788/
- Mahato M, et al. Nanoparticles for DNA delivery. Advances in Nanomedicine for the Delivery of Therapeutic Nucleic Acids 2017:59-81. https://www.sciencedirect.com/science/article/pii/B9780081005576000043
- Chen J, et al. Production and clinical development of nanoparticles for gene delivery. Molecular therapy Methods and Clinical Development 2016; Volume 3:16023. https://www.cell.com/molecular-therapy-family/methods/fulltext/S2329-0501(16)30165-6
- Tian H, et al. Nanoparticles for gene delivery. Small. 2013; 9: 2034-2044. https://www.ncbi.nlm.nih.gov/pubmed/23630123?dopt=Abstract
- Alvarez RD, et al. A phase II trial of intraperitoneal EGEN-001, an IL-12 plasmid formulated with PEG-PEI-cholesterol lipopolymer in the treatment of persistent or recurrent epithelial ovarian, fallopian tube or primary peritoneal cancer: a gynecologic oncology group study. Gynecol Oncol. 2014; 133: 433-438. https://www.gynecologiconcology-online.net/article/S0090-8258(14)00813-0/pdf
- Moran MC, et al. Gelatin-based nanoparticles as DNA delivery systems: Synthesis, physicochemical and biocompatible characterization. Colloids Surf B Biointerfaces. 2015;134:156-168. https://www.ncbi.nlm.nih.gov/pubmed/26188853/
- Peng LH, et al. TAT conjugated cationic noble metal nanoparticles for gene delivery to epidermal stem cells. Biomaterials 2014;35(21):5605-5618. https://www.ncbi.nlm.nih.gov/pubmed/24736021/
- Kim H, et al. Photothermally controlled gene delivery by reduced graphene oxide-polyethylenimine nanocomposite. Small. 2014 Jan 15; 10(1):117-126. https://www.ncbi.nlm.nih.gov/pubmed/23696272/
- https://aem.asm.org/content/aem/83/18/e00798-17.full.pdf
- https://www.ncbi.nlm.nih.gov/pmc/articles/PMC6073485/
- Nekkanti V, et al. Recent Advances in Liposomal Drug Delivery: A Review. Pharmaceutical Nanotechnology 2015;3(1):35-55 .DOI : 10.2174/2211738503666150709173905
- Candiani G, et al. Bioreducible Liposomes for Gene Delivery: From the Formulation to the Mechanism of Action. PLoS ONE 2010;5(10):e13430. https://doi.org/10.1371/journal.pone.0013430
- Templeton NS. Cationic liposome-mediated gene delivery in vivo. Biosci Rep 2002;22:283-95. https://www.researchgate.net/publication/11038132_Cationic_liposome-mediated_gene_delivery_in_vivo
- Majzoub RN, et al. Cationic liposome–nucleic acid nanoparticle assemblies with applications in gene delivery and gene silencing. Phil. Trans.R.Soc.A 2016;374:20150129. https://doi.org/10.1098/rsta.2015.0129
- Bombelli C, Faggioli F, Luciani P, Mancini G, Sacco MG (2005) Efficient transfection of DNA by liposomes formulated with cationic gemini amphiphiles. J Med Chem 48: 5378–5382.
- Candiani G, Frigerio M, Viani F, Verpelli C, Sala C, et al. (2007) Dimerizable redox-sensitive triazine-based cationic lipids for in vitro gene delivery. ChemMedChem 2: 292–296.
- Saffari M, Shirazi FH, Oghabian MA, Moghimi HR. Preparation and in-vitro evaluation of an antisense-containing cationic liposome against non-small cell lung cancer; a comparative preparation study. Iranian J. Pharm. Res. 2013;12 (Suppl.):3–10. https://www.ncbi.nlm.nih.gov/pmc/articles/PMC3813353/pdf/ijpr-12-03.pdf
- Dolatabadi JEN, et al. Solid Lipid Nanoparticles as Efficient Drug and Gene Delivery Systems: Recent Breakthroughs. Adv Pharm Bull. 2015;5(2):151–159. doi: 10.15171/apb.2015.022
- He SN, et al. Ternary nanoparticles composed of cationic solid lipid nanoparticles, protamine, and DNA for gene delivery. Int J Nanomedicine 2013;8:2859–2869. doi: 10.2147/IJN.S47967
- Shapiro G, et al. Multiparameter evaluation of in vivo gene delivery using ultrasound-guided, microbubble-enhanced sonoporation. J Control Release 2016;223:157. https://www.ncbi.nlm.nih.gov/pmc/articles/PMC4724495/
- Song S, et al. Explorations of high-intensity therapeutic ultrasound and microbubble-mediated gene delivery in mouse liver. Gene Therapy. 2011;18(10):1006–1014. https://www.ncbi.nlm.nih.gov/pmc/articles/PMC4549067/
- Fujii H, et al. Optimization of ultrasound-mediated antiangiogenic Cancer gene therapy. Molecular Therapy Nucleic Acids. 2013;2:e94. https://www.ncbi.nlm.nih.gov/pmc/articles/PMC4817934/
- Wu J, Li R-K. Ultrasound-targeted microbubble destruction in gene therapy: A new tool to cure human diseases Genes & Diseases 2017;4(2):64-74. https://www.sciencedirect.com/science/article/pii/S2352304216300344?via%3Dihub#bib23
- Huang C, et al. Advances in ultrasound-targeted microbubble-mediated gene therapy for liver fibrosis. Acta Pharmaceutica Sinica B 2017;7(4):447-452. https://www.sciencedirect.com/science/article/pii/S2211383516303045?via%3Dihub
- Hsu P-H, et al. Noninvasive and Targeted Gene Delivery into the Brain Using Microbubble-Facilitated Focused Ultrasound. PLoS ONE 2013;8(2): e57682. https://doi.org/10.1371/journal.pone.0057682
- Chen Z, et al. Recent Advances about Local Gene Delivery by Ultrasound. In Gene Expression and control 2018. https://www.intechopen.com/books/gene-expression-and-control/recent-advances-about-local-gene-delivery-by-ultrasound
- Horie S, Watanabe Y, Ono M, Mori S, Kodama T. Evaluation of antitumor effects following tumor necrosis factor-alpha gene delivery using nanobubbles and ultrasound. Cancer Science. 2011;102:2082-2089. doi: 10.1111/j.1349-7006.2011.02056.x
- Fan Z, et al. Mechanisms of microbubble-facilitated sonoporation for drug and gene delivery. TherDeliv. 2014;5(4):467–486. doi: 10.4155/tde.14.10
- Lundstrom K. Viral Vectors in Gene Therapy. Diseases 2018;6(2):42. doi: 10.3390/diseases6020042
- Garcia-Carbonero R, et al. Phase 1 study of intravenous administration of the chimeric adenovirus enadenotucirev in patients undergoing primary tumor resection. J Immunother Cancer 2017; 5(1):71.
- Spencer HT, et al. State of the art: gene therapy of haemophilia. Haemophilia 2016;22(5):66-71.
- Haworth KG, et al. CCR5-edited gene therapies for HIV cure: Closing the door to viral entry. Cytotherapy 2017;19(11):1325-1338. https://www.sciencedirect.com/science/article/abs/pii/S1465324917306205
- Goswami R, et al. Gene Therapy Leaves a Vicious Cycle. Front. Oncol., 24 April 2019. https://doi.org/10.3389/fonc.2019.00297
- https://pubs.rsc.org/en/content/articlehtml/2018/pp/c8pp00354h
- https://www.hindawi.com/journals/jnm/2019/4607531/
About BMG Labtech
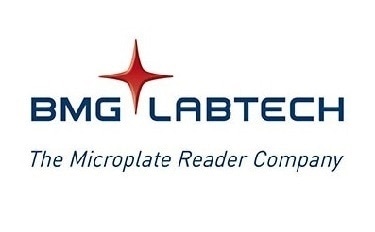
BMG LABTECH has been committed to producing microplate readers for more than twenty years. By focusing on the needs of the scientific community, the company’s innovative microplate readers have earned the company the reputation of being a technology leader in the field.
BMG LABTECH has developed a wide range of dedicated and multi-mode microplate readers for life sciences applications and high-throughput screening.
All BMG LABTECH microplate readers are "Made in Germany" and are conceived, developed, assembled, and tested entirely at our headquarters in Germany.
Since our establishment in Offenburg, Germany in 1989, BMG LABTECH has expanded to offer a worldwide sales and support network with offices in the USA, UK, Australia, Japan and France. Our subsidiaries, regional offices and distributors are committed to bringing you innovative microplate reader technology with the quality and reliability you expect from a German company.
Our staff includes engineers and scientists from the fields of biology, biochemistry, analytical chemistry, and physics.
Sponsored Content Policy: News-Medical.net publishes articles and related content that may be derived from sources where we have existing commercial relationships, provided such content adds value to the core editorial ethos of News-Medical.Net which is to educate and inform site visitors interested in medical research, science, medical devices and treatments.