Introduction
To study neurological diseases, a major limitation is the ability to culture, observe, and study neuronal cells that accurately characterize human phenotypes neurological disorders. A new approach has been developed by employing human induced pluripotent stem cell (hiPSC)-derived neurons to model neurological diseases.
To characterize and evaluate such novel model systems, it is important to monitor cell health and neuronal morphology in long-term cultures.
Traditional methods are based on endpoint assays and imaging methods involving the use of immunochemical staining, which does not have real-time kinetic information. Hence, when nervous system models are studied utilizing these traditional methods, the dynamic complexity of neurological features and responses is lost, and the neural network integrity is compromised.
Continual real-time monitoring is advantageous to study these model systems as it delivers a more physiologically relevant picture of neuronal cell behavior. This enables the same neuronal networks to be measured repeatedly, over time, in a non-invasively manor, allowing rare or transient events to be captured. Such events cannot be captured with end-point assays.
This article describes real-time methods and presents validation data that highlights optimal culture conditions for evaluating neurite outgrowth and cell viability in hiPSC-derived neurons from Cellular Dynamic International (CDI, iCell Neurons).
Cellular viability and neurite outgrowth in iCell Gluta Neurons from CDI are monitored using a quantitative, live-cell imaging and analysis method with the IncuCyte® S3 over days/weeks in 96-well microplate culture.
Here, neuronal excitotoxicity is assessed using the IncuCyte® S3 Phase/Fluorescent NeuroTrack applications multiplexed with Annexin V reagents to demonstrate a real-time imaging and analysis method using hiPSC-derived neurons. These assays highlight optimal culture conditions for an iPSC-derived neuronal system and illustrate the capability of the IncuCyte method for real-time, long-term quantitative analysis of iPSC-derived neuronal cell health.
Materials and methods
Optimization of culture conditions
After coating 96-well cell culture plates (MidSci, #TPP92096) with 100 μg/mL poly-D-lysine (PDL, Millipore, A-003-E), 0.01% poly-L-ornithine (PLO, Sigma, P6355) or 0.07% polyethylenemine (PEI, Sigma, 408727), they were incubated at room temperature overnight in a tissue culture hood. Surface coatings were totally aspirated after incubation, followed by rinsing each well two times with 150 μL WFI water.
Then, plates were allowed to dry with the lid removed for at least one hour in a tissue culture hood. 0.028 mg/mL Matrigel or 3.3 μg/mL laminin was added to wells with additional laminin/Matrigel coating and incubated for at least 1 hour at 37°C. To avoid drying, Matrigel and laminin solutions were immediately aspirated before adding cell suspension.
Barring culture surface coatings, iCell Neurons were handled, stored, and preserved in accordance with manufacturer’s instructions described in CDI’s iCell Neurons User’s Guide. Next, these iCell Neurons were seeded at 10,000 or 50,000 cells per well to assess excitotoxicity or coatings, respectively. The IncuCyte S3® live cell imaging and analysis system was used to monitor neurite outgrowth and cellular morphology for a period of 14 days.
To perform co-culture experiments, IncuCyte® NeuroLight Red (MOI 1) was used to infect iCell Neurons 2 to 4 hours after plating. After 16 to 24 hours of incubation, virus was taken out and media were substituted, and this was followed by seeding primary rat astrocytes at 15,000 cells per well.
On the third day of post-plating, half of the media was replaced with media comprising of uridine and 5-fluoro-2’-deoxyuridine (28 μg/mL and 8 μg/mL, respectively) to prevent further proliferation of astrocytes.
Evaluation of neuronal excitotoxicity
0.07% PEI + 3.3 μg/mL laminin, was used to coat 96-well cell culture plates. iGluta Neurons were handled, stored, and preserved in accordance with the manufacturer’s instructions described in CDI’s iCell GlutaNeurons User’s Guide; penicillin/streptomycin and laminin were however excluded from the media. After seeding the cells at 10,000 cells per well, neurite outgrowth and cellular morphology were observed for a period of 14 days through the IncuCyte S3 live-cell imaging and analysis system.
To perform co-culture experiments, astrocytes were seeded, IncuCyte NeuroLight Red was used to infect the neurons, and cells were treated. On DIV14, cells were subjected to kainate +/- glutamate or glutamate receptor antagonists in the presence of green or red IncuCyte® Annexin V reagent, which is a fluorescent marker of cell apoptosis or death. The IncuCyte S3 live-cell analysis platform in tandem with the IncuCyte® NeuroTrack analysis software was used to monitor and measure the neurite length and apoptosis for a period of 72 hours.
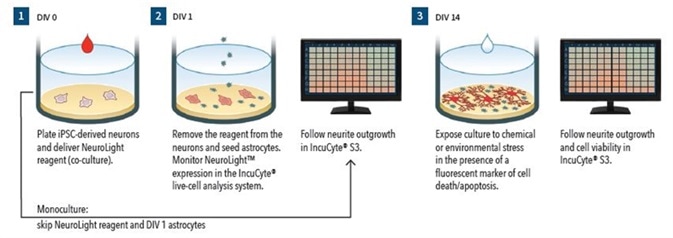
Figure 1.
Assay Workflow (Monoculture):
- DIV0 – Wells are coated and iPSC-derived neurons are plated
- Neurite outgrowth is monitored using Phase NeuroTrack
- DIV14 - In the presence of Annexin V red reagent (apoptosis marker), cells are treated with neurite and cell health modulating test agents
- The IncuCyte S3 is used for 72 hours to follow cell viability and neurite outgrowth
- Assay Workflow (Co-culture)
- DIV0 – Wells are coated, iPSC-derived neurons are plated, and IncuCyte NeuroLight reagent is delivered
- DIV1 – Virus is removed and primary rat astrocytes 15K cells/well are plated.
- DIV3 - 50% media are removed and replaced with media comprising of uridine and 5-fluoro-2’-deoxyuridine
- Neurite outgrowth is tracked using IncuCyte Fluorescent NeuroTrack
- DIV14 – In the presence of IncuCyte Annexin V green reagent (apoptosis marker), cells are treated with neurite and cell health modulating test agents
- Neurite outgrowth and cell viability are followed using the IncuCyte S3 for a period of 72 hours
Validation data
Culture plate coating conditions
While hiPSC technology has become a viable model for investigating diseases of the nervous system in humans, the necessary culture conditions for plating and preserving these cells has not been fully defined. In this study, three typically used culture substrates +/- secondary Matrigel or laminin coating were tested to find out the optimal conditions for in vitro neurite outgrowth and cellular morphology in neurons derived from hiPSC.
Shown in Figure 2 are 10x high definition phase images obtained on DIV14 of iCell Neurons that were seeded at 50,000 cells per well in culture plates, coated with PLO, PDL, or PEI +/- Matrigel or laminin. PLO- or PDL-coated plates were found to have remarkable radial neurite cabling and cell clustering, which could be because neurons adhere suboptimally to the plate. Irrespective of secondary coating with Matrigel or laminin, this clustering morphology took place on PLO- or PDL-coated plates.
However, iCell Neurons seeded on PEI-coated plates exhibited a cell monolayer that was more uniform and had typical neurite outgrowth. Although neurite outgrowth seemed to be more robust, a similar neuronal monolayer is shown as PEI alone by secondary coating with Matrigel or laminin.
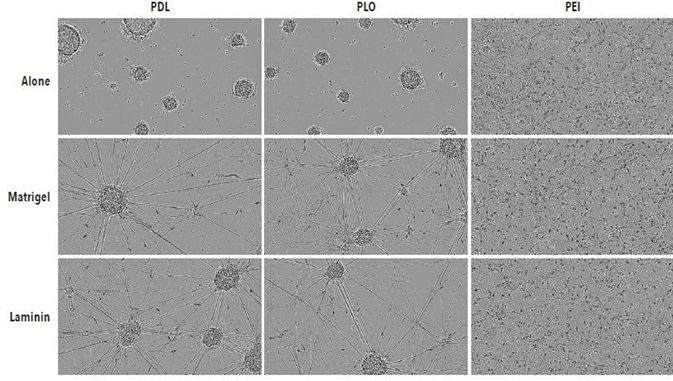
Figure 2. Optimal adherence and morphology of iCell Neuron monocultures seeded on PEI + laminin. iCell Neurons were seeded at 50,000 cells/well on PDL, PLO or PEI +/- Matrigel or +/- laminin. Cells plated on PDL or PLO formed large neurospheres by DIV14 in the presence or absence of additional laminin or Matrigel coating. Cells plated on PEI +/- laminin or Matrigel displayed a more homogenous monolayer. All images captured at 10x magnification.
Since similar neurite outgrowth and cellular morphology were shown by secondary coating with Matrigel or laminin after PEI coating, laminin was used for additional experimentation as it was relatively easy to use. To find out whether laminin-based secondary resulted in a statistically notable increase in neurite outgrowth as opposed to PEI alone, the Phase NeuroTrack software was used on the IncuCyte S3 system to measure the length of neurite over a given time for both culture conditions.
20x phase images of iCell Neurons, which were seeded in PEI + laminin or PEI alone at DIV3 and DIV14, are shown in Figure 3. Compared to cells grown on PEI alone, cells grown on PEI + laminin at DIV3 and DIV14 showed distinctly improved outgrowth of neurites. The Phase NeuroTrack analysis software was used on the IncuCyte S3 system to measure and graph this increase over time.
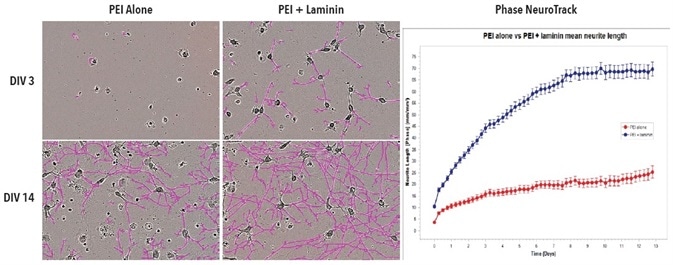
Figure 3. Improved neurite outgrowth in iCell Neuron monoculture seeded on PEI + laminin compared to PEI alone. Cell culture plates were coated with PEI or PEI + laminin. iCell Neurons were seeded at 10,000 cells/well. Phase images show neurite length at DIV3 and DIV14. Neurite length was quantified using Phase NeuroTrack software and plotted over time. All images captured at 20x magnification. Each data point represents mean +/- SEM, n=4.
Test was done to find out if iCell Neurons’s optimized seeding conditions in monoculture on PEI + laminin converted to iCell Neurons that were plated in co-culture with primary rat astrocytes. Shown in Figure 4 are fluorescent and phase images of iCell Neurons that were plated on PEI + laminin in co-culture with primary rat astrocytes at DIV14 and DIV6.
During the experiment, a robust increase was observed in the neurite length eventually without any clustering of astrocytes or neurons at any point. While iCell Neurons in co-culture with primary rat astrocytes did not show a major difference in neurite length or cellular morphology with other coating conditions (data not shown), seeding on PEI + laminin is recommended to sustain consistency with monoculture experiments.
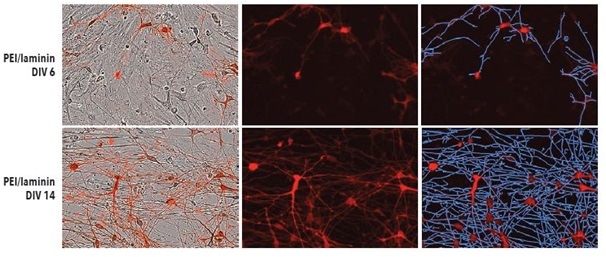
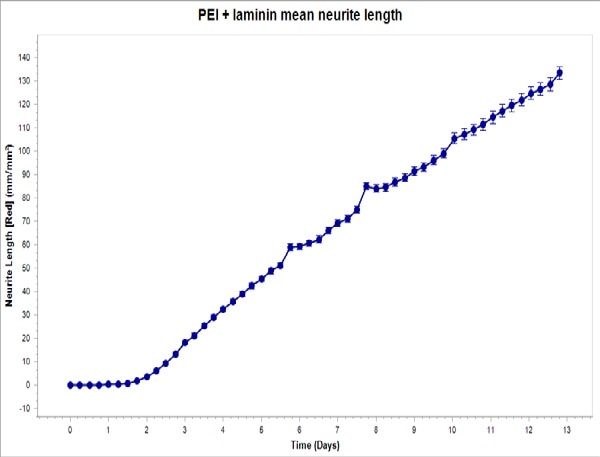
Figure 4. Top: PEI + laminin coating enables robust neurite outgrowth in iCell Neuron co-culture with primary rat astrocytes. Cell culture plates were coated with PEI + laminin. iCell Neurons were seeded at 10,000 cells/well and primary rat astrocytes were seeded at 15,000 cells/well. Bottom: iCell Neurons were infected with IncuCyte NeuroLight Red and neurite length was quantified using Fluorescent NeuroTrack software and plotted over time. All images captured at 20x magnification. Each data point represents mean +/- SEM, n=4.
Analysis and quantification of neuronal excitotoxicity
To study the cellular mechanisms underlying neurotoxicity and neurological disorders, in vitro models of human neurophysiology are required. In 2007, Conforti et al. demonstrated that the length of the neuritis can be employed as a sensitive marker of neurotoxicity and neurodegenerative disease. It has also been demonstrated that neurotransmitter-mediated induced toxicity including surplus glutamate is associated with certain forms of disease progression (Choi, 1988; Mattson, 2008).
Using in vitro and in vivo non-human cells/subjects, present-day studies on glutamate and other neurotransmitter-induced neurotoxicity are frequently carried out, but these efforts are made more complicated by the high level of interspecies variations when attempts are made to model human disease. Further, short term readouts are used by many in vitro models that may find it difficult to identify chronic toxicity, whereas in vivo models provide only limited throughput.
In this analysis, fluorescent (co-culture) and HD phase (monoculture) NeuroTrack analysis software was used that was multiplexed with IncuCyte Annexin V cell viability reagents to quantitatively define possible long term neurotoxic effects of kainate and glutamate in neurons derived from hiPSC.
Typical phase images of iGluta Neurons in monoculture at DIV17, DIV14, and DIV2 are shown in Figure 5. After image acquisition, glutamate (1 mM) was added on DIV14 and its impact on cell viability and neurite length was observed for a period of 72 hours. At DIV17, neurite length was significantly reduced after the addition of glutamate.
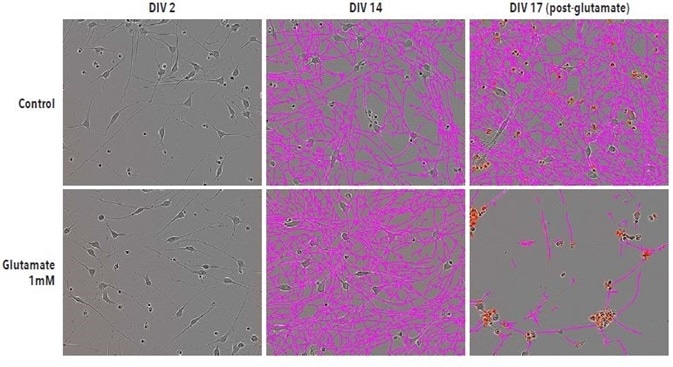
Figure 5. Glutamate-induced excitotoxicity reduces neurite length and increases cell death. Example Phase NeuroTrack images for quantifying neurite length following excitotoxic agent administration in iGluta neurons imaged at DIV2, DIV14 and DIV17. DIV17 corresponds to 72 hrs post-glutamate (1mM) addition. Neurite length is significantly reduced following glutamate treatment. A corresponding increase in IncuCyte Annexin V (red) fluorescence indicates an increase in cell death. All images captured at 20x magnification.
When both kainate and glutamate were added at DIV14, it resulted in a time- and concentration- dependent decrease in the length of neurites with associated increase in cell apoptosis/death in iGluta Neurons in both co-culture and monoculture with primary rat astrocytes, as shown in Figures 6 to 9.
In order to examine a possible mechanism that mediates this toxicity, MK801 and NBQX (NMDA receptor and AMPA receptor antagonists, respectively) were added at DIV14 together with kainate or glutamate. It was observed that MK801 and NBQX decreased the neurotoxic effects of kainate and glutamate, indicating that these neurotoxic effects are mediated by glutamate receptor to some extent.
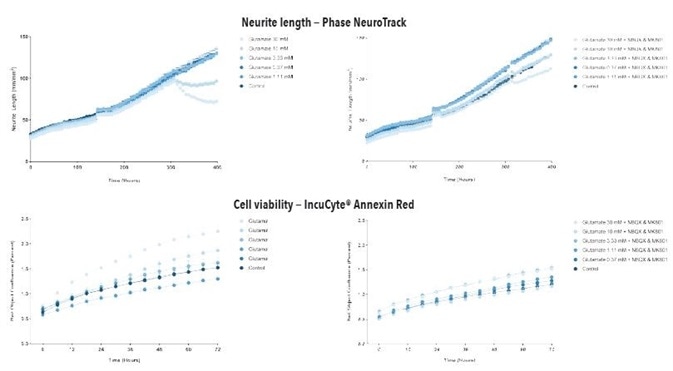
Figure 6. Glutamate exposure decreases neurite length and increases cell death in a concentration- and time-dependent manner in iGluta Neuron monoculture. Time course of the effects of glutamate addition at day 14 (arrow) on neurite length (top panels) and cell viability (bottom panels). Glutamate causes a concentration-dependent decrease in neurite length with concomitant decrease in cell viability (increased IncuCyte Annexin Red fluorescence). Addition of NBQX (20 μM) & MK801 (10 μM) protects iGluta Neurons from glutamate toxicity. Each data point represents mean +/- SEM, n=6.
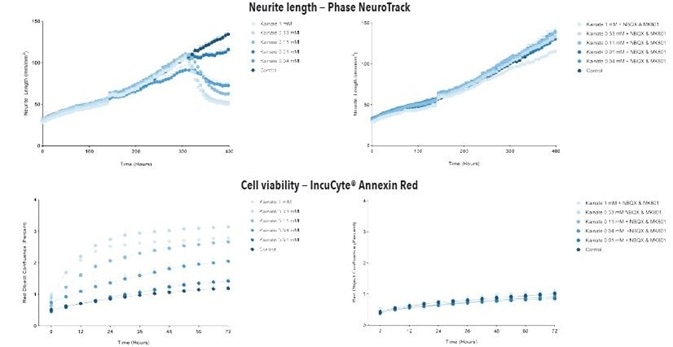
Figure 7. Kainate exposure decreases neurite length and increases cell death in a concentration- and time-dependent manner in iGluta Neuron monoculture. Time course of the effects of kainate addition at day 14 (arrow) on neurite length (top panels) and cell viability (bottom panels). Kainate causes a concentration-dependent decrease in neurite length with concomitant decrease in cell viability (increased Annexin Red fluorescence). Addition of NBQX (20 μM) & MK801 (10 μM) protects iGluta Neurons from kainate toxicity. Each data point represents mean +/- SEM, n=6.
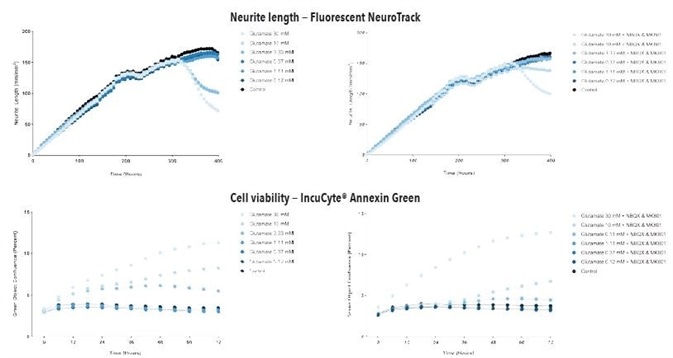
Figure 8. Glutamate exposure concentration- and time-dependently decreases neurite length and increases cell death in iGluta Neuron co-culture. Time course of the effects of glutamate addition at day 14 (arrow) on neurite length (top panels) and cell viability (bottom panels). Glutamate causes a concentration-dependent decrease in neurite length with concomitant decrease in cell viability (increased Annexin Green fluorescence). Addition of NBQX (20 μM) & MK801 (10 μM) protects iGluta Neurons from glutamate toxicity. Each data point represents mean +/- SEM, n=6.
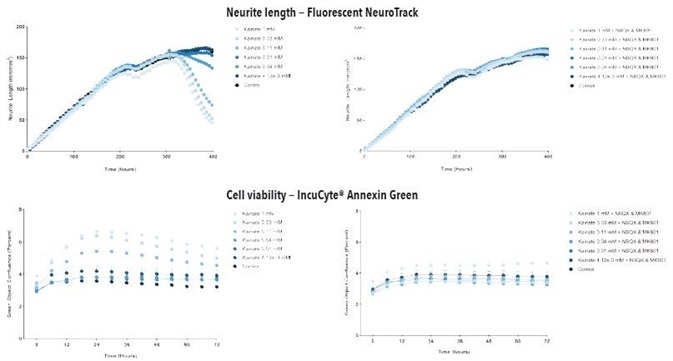
Figure 9. Kainate exposure concentration- and time-dependently decreases neurite length and increases cell death in iGluta Neuron co-culture. Time course of the effects of kainate addition at day 14 (arrow) on neurite length (top panels) and cell viability (bottom panels). Kainate causes a concentration-dependent decrease in neurite length with concomitant decrease in cell viability (increased Annexin Green fluorescence). Addition of NBQX (20 μM) & MK801 (10 μM) protects iGluta Neurons from kainate toxicity. Each data point represents mean +/- SEM, n=6.
Conclusion
When testing plate coating and multiple culture conditions, it was discovered that iCell Neurons optimally adhere with a laminin or PEI plate coating. To demonstrate a real-time imaging method through hiPSC-derived neurons, the IncuCyte S3 phase and fluorescent NeuroTrack applications - multiplexed with Annexin V reagents in iCell Gluta Neurons - were used to evaluate the excitotoxicity induced by kainate and glutamate.
Both kainate and glutamate led to a time- and concentration-dependent decrease in the length of neurites with an associated increase in green or red object count (suggesting cell death/apoptosis) over a period of 72 hours. The effects of glutamate and kainate on neurite length and cell apoptosis were reduced by treatment with the AMPA receptor antagonist NBQX and the NMDA receptor antagonist MK-801.
Based on these detailed results, it is seen that the IncuCyte S3 Live-Cell Analysis system can be used to analyze neurotoxicity in a hiPSC-derived neuron model over days or even weeks in culture. The system provides automated analysis of microplate assays, allowing users to improve the culture conditions and maintain their own models of iPSC-derived neurons.
Using the IncuCyte NeuroTrack software, iPSC-derived neurons can be imaged in co-culture or in monoculture with astrocytes and real-time changes in cell viability and neurite length can be quantitated. Also, this method offers a sensitive way for detecting pharmacological manipulations that modify the dynamics of neurites and also induce toxicity.
The IncuCyte method for long-term and real-time quantitative assessment of neuronal cell health meets the crucial requirement in the analysis of iPSC-derived neurons and human neurophysiological disorders.
References
- Conforti L, Adalbert R and Coleman MP: Neuronal death: where does the end begin? Trends Neurosci 2007, 30:159-66.
- Choi DW: Glutamate neurotoxicity and diseases of the nervous system. Neuron 1988, 1:623-34.
- Mattson MP: Glutamate and neurotrophic factors in neuronal plasticity and disease. Ann N Y Acad Sci 2008, 1144:97-112.
Sartorius
Sartorius is a leading international pharmaceutical and laboratory equipment supplier. With our innovative products and services, we are helping our customers across the entire globe to implement their complex and quality-critical biomanufacturing and laboratory processes reliably and economically.
The Group companies are united under the roof of Sartorius AG, which is listed on the Frankfurt Stock Exchange and holds the majority stake in Sartorius Stedim Biotech S.A. Quoted on the Paris Stock Exchange, this subgroup is comprised mainly of the Bioprocess Solutions Division.
Innovative Technologies Enable Medical Progress
A growing number of medications are biopharmaceuticals. These are produced using living cells in complex, lengthy and expensive procedures. The Bioprocess Solutions Division provides the essential products and technologies to accomplish this.
In fact, Sartorius has been pioneering and setting the standards for single-use products that are currently used throughout all biopharmaceutical manufacturing processes.
Making Lab Life Easier
Lab work is complex and demanding: Despite repetitive analytical routines, lab staff must perform each step in a highly concentrated and careful way for accurate results.
The Lab Products and Services Division helps lab personnel excel because its products, such as laboratory balances, pipettes and lab consumables, minimize human error, simplify workflows and reduce physical workloads
Sponsored Content Policy: News-Medical.net publishes articles and related content that may be derived from sources where we have existing commercial relationships, provided such content adds value to the core editorial ethos of News-Medical.Net which is to educate and inform site visitors interested in medical research, science, medical devices and treatments.