An increasing body of research indicates that more translational and relevant evaluations can be made utilizing 3D organoids and micro-tissues as opposed to 2D monolayer cell models. This is most widespread in the study of hepatotoxicity, cancer biology, and immuno-oncology.1
While affordable, 2D models fail to reflect the complexity of an in vivo tumor compared to 3D models and the related effects of the tumor microenvironment, for example the effect of the extracellular matrix (ECM) and that of cell-to-cell contact.2
For instance, in comparison to 2D cultures, 3D tumor spheroids display increased cell survival and more relevant morphology.
Spheroid models contain a layered structure including an outer layer of more quickly proliferating cells, and a quiescent zone and hypoxic necrotic core caused by a gradient of oxygen, nutrients, and metabolites.
These are crucial features for the assessment of drug resistance caused by the effects of penetration in a heterogeneous tumor.2,3
Single spheroids created in ultra-low attachment (ULA) plates are ideally suited to scientists aiming to assess larger solid tumors, which may contain hypoxic cores, and have a need for high degrees of well-to-well consistency. This method also excludes the use of poorly characterized and complex biomatrices.
There are a limited number of modern techniques for evaluating the shrinkage and growth of 3D tumor spheroids. The techniques involve one or more of the following:
- Assay workflows that are laborious, time-consuming, or expensive.
- An instruction to label the cells (for example a fluorescent probe) which can disrupt the biology and may not be responsive to primary tissue.
- Single time point readouts that do not describe the complete duration.
- When cells are removed from the incubator for imaging, the environmental control is interrupted.
- Indirect readouts (for example ATP) which can miss important morphological data and/or inaccurately describe cell growth.
Assay principle
In this white paper, the validation and methods for miniaturized (96/384-well) Incucyte® S3 Single Spheroid Assays based on non-invasive bright-field image analysis, using the Incucyte® S3 Spheroid Software module are described.
DF Brightfield, the Incucyte proprietary image acquisition method, was utilized to take extended depth of focus, high contrast images in combination with the Incucyte spheroid assay protocol.
Continual analysis and segmentation were carried out with an automated image processing algorithm for the generation of data, which decreased operator bias and covered the biggest BF object in the field. This allowed for the evaluation of spheroid viability with no requirement for invasive reagents.
These assays were simple to run, dynamic, utilized validated and non-invasive reagents, and delivered automated imaging to observe morphological differences and direct measurement of tumor health and size in real time.
Formed in ULA plates, tumor spheroids were moved inside the incubator to uphold physiological conditions and were observed for up to two weeks to observe kinetic variations.
As outlined here, fluorescence characterization was attained by identifying fluorescence inside a Brightfield mask boundary, excluding the need to establish fluorescence masking parameters, for example threshold setting.
The software analysis tools allowed robust data and objective analysis appropriate for pharmacological evaluation. Pharmacological utility was additionally explored, and involved proven spheroid shrinkage and growth assays with strong intra- and inter-plate reproducibility.
Cellular responses to both cytostatic (cycloheximide) and cytotoxic (camptothecin) agents were evaluated in cells stably expressing Incucyte® CytoLight/NucLight fluoroprobes or cell health reagents (IncuCyte® Caspase 3/7 or Annexin V) in order to demonstrate proof of concept for exploring the mechanisms of drug action.
Taken together, the use of the Incucyte S3 Single Spheroid Assays, Incucyte reagents, with the Incucyte® S3 Spheroid Software Module offer a more thorough solution to solve the current challenges of 3D spheroid models, enabling real time kinetic evaluation of the shrinkage and growth of 3D tumor spheroids with stronger physiological relevance.
This allows increased model understanding for the determination of drug targets, along with the optimization of pharmacological interpretation and analysis.
Materials and methods
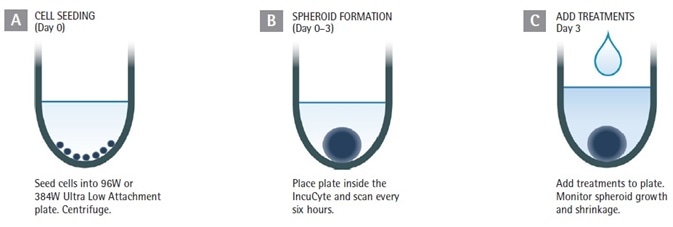
Figure 1. Assay Workflow. Image Credit: Sartorius
1. Cells of interest are counted, harvested, and plated onto ULA round-bottom 384- or 96-well plates at the required densities (in 50 or 100 µL per well for 384- or 96-well plate respectively).
The plates are then centrifuged (150 xg, 10 minutes). The seeding density must be optimized for every cell line employed; suggested range of 1,000 to 5,000 cells per well (10,000 to 50,000 cells per mL seeding stock).
2. The creation of the spheroid is observed to the target size (such as 200 to 500 µm in diameter) with Brightfield and HD phase-contrast image acquisition (either 4x or 10x magnification) every 6 hours utilizing the Incucyte® S3 Live Cell Analysis System.
3. Optional: Incucyte® Cell Health reagents can be added up to three days after seeding or at the formation.
4. Inclusion of test compounds (25 µL at 3x, or 100 µL at 2x final assay concentration (FAC) per well for 384- or 96-well plate respectively).
5. The spheroid shrinkage and growth assay is generated and tracked in Incucyte (6 hours of repetitive scanning, for up to two weeks). The size of the tumor is presented in real time according to the Brightfield image analysis.
Unless otherwise stated, all cell culture reagents were acquired from Life Technologies. SKOV3 (EACC) cultures were stably transfected with Incucyte® NucLight Red Lentivirus, Puromycin selection, EF1 Alpha Promoter, #4625, prepared according to Essen BioScience protocol.
Incucyte® NucLight Red Cellular Reagents (HT-1080-NR, #4485, A549-NR #4491, MDA-MB-231-NR,#4487) and SKOV3-NR cultures were developed to confluence in 75 cm2 tissue culture treated flasks and seeded into 96-well (Corning, #7007) or 384-well ULA round-bottom plates (S-Bio, PrimeSurface® 3D Spheroid Plates, #MS-9384UZ).
Spheroids grew to the target size after 72 hours. The plates were then centrifuged (150 xg) at room temperature for 10 minutes. Matrigel® Basement Membrane Matrix (Corning #356234) at 2.5% v/v was required for the MDA-MB-231 cells to enhance tight spheroid formation.
Each of the cells were cultured in F-12K supplemented with 0.5 µg/mL puromycin, 1% glutamax, 1% Pen/Strep, and 10% FBS. The creation of spheroids was observed in an IncuCyte throughout a 72-hour period at intervals of 6 hours.
Where necessary, Incucyte® Cell Health reagents were introduced to establish cytotoxicity (Incucyte® Cytotox Red or Green, EssenBio #4633 or #4632), Caspase 3/7 activity (Incucyte® Caspase 3/7 Green or Green, EssenBio #4440 or #4704) or phosphatydil serine externalization (Incucyte Annexin V Red or Green, EssenBio #4642 or 4641).
Validation data
Spheroid morphology
Breast tumor (MDA-MB-231 in Matrigel) and human lung (A549) cell lines were seeded at 2,500 or 5,000 cells per well respectively in 96-well ULA plates (Corning) to demonstrate the value of the Incucyte® imaging solution.
High definition (HD)-phase and Brightfield (BF) images were captured at intervals of 6 hours (Figure 2). Distinct morphological variations were observed: MDA-MB231 spheroids were compact and small, whereas A549 cells produced larger, loose aggregates. These characteristics are in line with those previously outlined elsewhere.
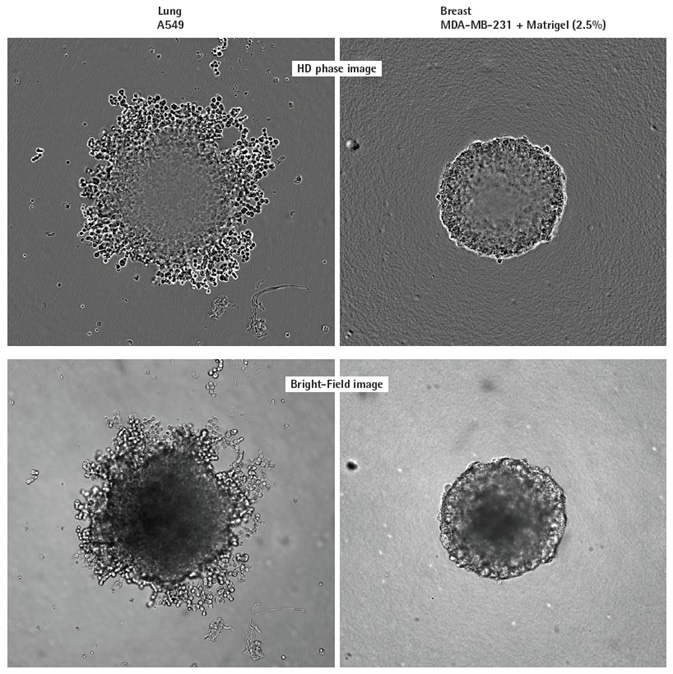
Figure 2. Visualizing different spheroid morphologies. High quality HD phase and corresponding BF images of spheroids formed from A549 and MDAMB-231 cells (2,500 or 5000 cells per well respectively), 72 h post seeding. Visualization of detailed phenotypic variation is observed in HD phase images. A549 cells present a loose aggregate morphology compared to the compact spheroid formed by MDA-MB-231 cells. Compaction of MDA-MB-231 aggregates into spheroids was achieved by the addition of 2.5% v/v Matrigel® post centrifugation. All images captured at 10x magnification. Image Credit: Sartorius
Label-free kinetic monitoring of spheroid growth
To verify the capabilities for kinetic quantification, the tumor spheroid size was calculated over time utilizing the Incucyte® S3 DF Brightfield analysis automated software algorithm that covered the biggest Brightfield object in the field of view.
Variations in the morphology and size of A549, HT-1080, and MDA-MB-231 tumor spheroids were tracked over time for the production of growth curves, in the presence and absence of the cytotoxic drug, camptothecin (CMP; Figure 3).
The control spheroids significantly increased during the 10-day period (2-8 fold) for all three cell types. The greatest increase in size was seen with MDA-MB-231 in Matrigel. CMP limited the growth of spheroids, and in the example of HT-1080 cells, a total decrease in spheroid size in comparison with the t= zero control was noted.
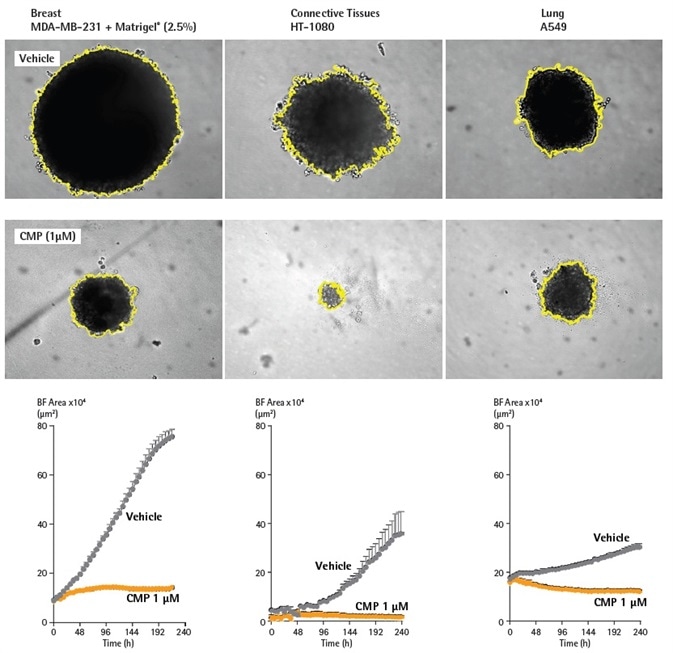
Figure 3. BF analysis enables accurate kinetic quantification of spheroids. The differential pharmacological effect of 1 µM CMP on growth of MDA-MB-231, HT-1080 and A549 cells in a 3D spheroid assay. Cells were grown in ULA round-bottom 96-well plates (2,500 cells per well) for 72 h and treatment with ± 1 µM CMP followed. Segmented Brightfield images compare treated vs. untreated conditions at 240 h. Time courses illustrate the specific cell type-dependent kinetic profile of spheroid growth and shrinkage. The graphs display the Largest Brightfield Object area (µm2) (y-axis) over the course of a 240 h assay (x-axis) at 6 h intervals. All images captured at 10X magnification. Each data point represents mean ±SEM, n=4. Image Credit: Sartorius
Demonstration of pharmacological utility
96-well spheroid growth and shrinkage assay
To demonstrate the suitability of this technique for the testing of drug toxicity, a pharmacological investigation was carried out in the SKOV3 ovarian cancer cell line (Figure 4).
For 72 hours, cells were developed in ULA round-bottom 96-well plates and were then treated with either the chemotherapeutic drug oxaliplatin (OXA), the cytotoxic compound CMP, or the apoptotic compound cisplatin (CIS).
The Incucyte® was used to measure the effects on spheroid shrinkage and growth by the assessment of variations in spheroid area utilizing the Largest Brightfield Object Area (µm2) metric.
For all compounds, a concentration dependent inhibitory growth effect was seen which shows how the potencies of compounds can be directly contrasted within the same assay.
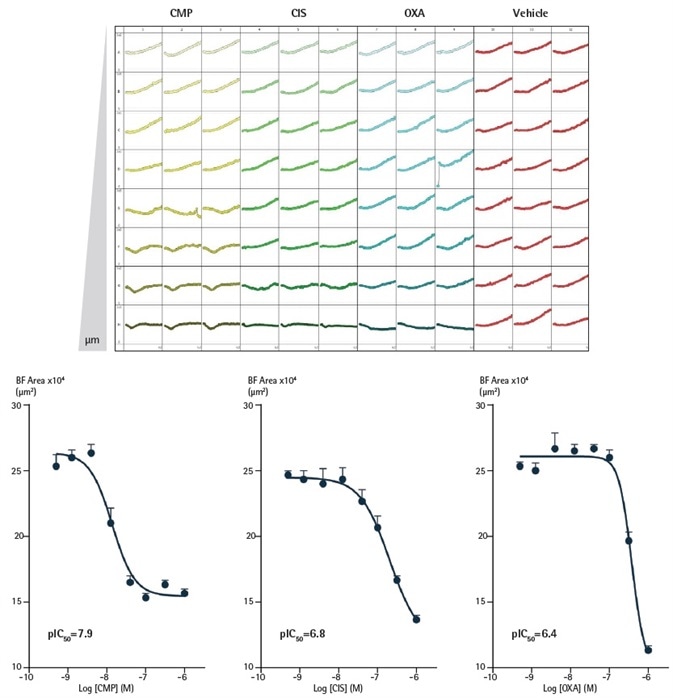
Figure 4. Effect of CMP, CIS and OXA on growth of SKOV3 cells in a 3D spheroid assay. SKOV3 cells were plated at a density of 5,000 cells/well and spheroid allowed to form (72 h). Cells were then treated with serial compound dilutions and kinetics of spheroid growth and shrinkage were obtained. Plateview shows the individual well Largest Brightfield area (µm2) over time. Concentration response curves represent the Largest Brightfield area (µm2) at 204 h post-treatment. Data were collected over 240 h period at 6 h intervals. Each data point represents mean ±SEM, n=8. Image Credit: Sartorius
Miniaturizing spheroid growth and shrinkage assay with robust intra- and inter-plate reproducibility
To enhance data throughput and output, the 3D spheroid shrinkage and growth assay was miniaturized to 384-well format. SKOV3, A549, and HT-1080 cell types were investigated at four seeding densities (Figure 5).
For 72 hours, t cells were plated in 384-well ULA round-bottom plates, and the Brightfield area of the spheroids was measured on the Incucyte® system utilizing the Largest Brightfield Object Area (µm2) metric.
The results show the differential growth profile and cell area dependence with seeding density throughout all cell types. Utilizing the investigation outlined above, both the intra- and interplate reproducibility was evaluated.
As demonstrated, the size of the spheroids was dependent on the seeding density, and the cell growth profiles correlated with differential growth kinetics. Inter-plate variability analysis was executed at a 2,500 cells per well seeding density. Reproducible Brightfield area metrics were acquired for all plates for both CMP and vehicle-treated spheroids.
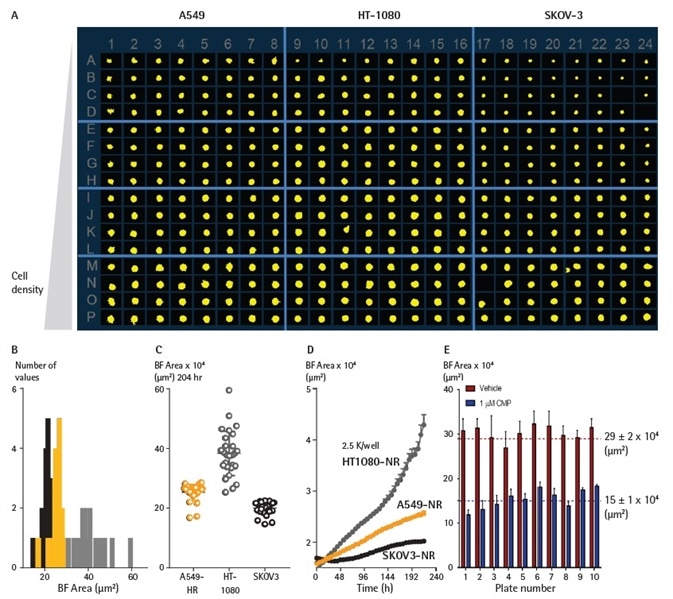
Figure 5. Miniaturizing spheroid growth and shrinkage assay for assay optimization. Comparison of temporal growth profiles of A549, HT-1080 and SKOV3 cells in a miniaturized 3D spheroid assay. (A) All cells seeded at a density ranging from 310 to 7,500 cells per well plated in a ULA round-bottom 384-well plate. Media was replenished 72 h post seeding. (A) Microplate overview image shows Brightfield segmentation mask at 204 h post-media replenishment. (B) Histogram compares the distribution frequency of the Brightfield area (µm2) across all cell types plated at 2,500 cells/well at this time-point. (C) Variability plot analysis shows the largest Brightfield of individual wells at 204 h. (D) Time course plots represent the differential temporal profile of the Largest Brightfield Object Area metric (µm2) across the cell types. Data were collected over a 204 h period at 6 h intervals, all images captured at 10x magnification. Each data point represents mean ±SEM, n=32. Reproducible BF area metrics across plates for vehicle- and CMP-treated spheroids (D) BF area metrics were reproducible across plates for both vehicle- and CMP-treated spheroids. Image Credit: Sartorius
Investigation of drug mechanisms of action with viability and toxicity measurements
A readout of cell viability is provided by the expression of fluorescent proteins inside the cells of a spheroid. A loss of fluorescence signal is caused by cytotoxic challenge, whereas spheroid growth results in an increase in fluorescence. The object of interest can be identified through the masking of the Brightfield channel.
Using the ‘fluorescence within the Brightfield boundary’ feature in the Incucyte® S3 Spheroid Module enables the identification of the mean fluorescence intensity of the pixels comprised within the object of interest.
The integrated fluorescence intensity inside the Brightfield boundary delivers an analysis appropriate for identifying both the death and growth of a spheroid from a single readout.
The impact of CMP towards cell viability was investigated in SKOV3 cells which were stably expressing a red fluorescent protein (NucLight Red). The cells created compact spheroids with equivalent features to the wild-type cells established on Brightfield analysis (data not shown).
After the three-day formation stage, fluorescent measurements (integrated intensity inside the Brightfield boundary) were calculated (Figure 6 below). The fluorescence intensity increased in proportion to the increase in spheroid size in vehicle-treated spheroids.
The introduction of CMP (1 µM) generated a significant decrease in fluorescence, reaching background levels following 192 hours. As outlined previously, the fluorescence within a Brightfield boundary analysis removed the requirement to apply a fluorescence mask, and also negated the effect of threshold settings.
The functions of cell viability can additionally be investigated by utilizing Incucyte® Cell Health reagents with spheroids (Annexin V Green/Red, EssenBio #4641/#4642 or Cytotox Green/ Red, EssenBio #4632/#4633).
The introduction of phosphatidyl serine externalization markers and Incucyte® Annexin V reagents, at the point of formation or up to 96 hours after formation, allows for the identification of apoptosis levels inside the spheroid.
Incucyte® Cell Health Reagents were utilized to establish the function of cell death following treatment with CMP (Figure 7). In the presence of formed compact spheroids and Incucyte® Annexin V Apoptosis Green reagents (EssenBio #4642), A549 cells (human lung carcinoma line) were seeded at 2,500 cells per well.
The size of spheroids was observed employing Brightfield masking, which demonstrated a moderate size increase during 10 days of vehicle-treatment. The inclusion of camptothecin (0.5 nM to 1 µM) yielded a concentration-dependent decline in spheroid growth, stopping an increase in size at the highest concentration tested.
Little to no depletion in the size of the spheroids was found. Fluorescence analysis showed an equivalent concentration-dependent influence with increases in the mean intensity inside the Brightfield boundary, indicating the generation of apoptosis.
The plotting of concentration response curves for Brightfield and mean fluorescence offered equivalent IC50/EC50 values; 10.8 nM for the apoptotic readout and 4.0 nM for the size analysis.
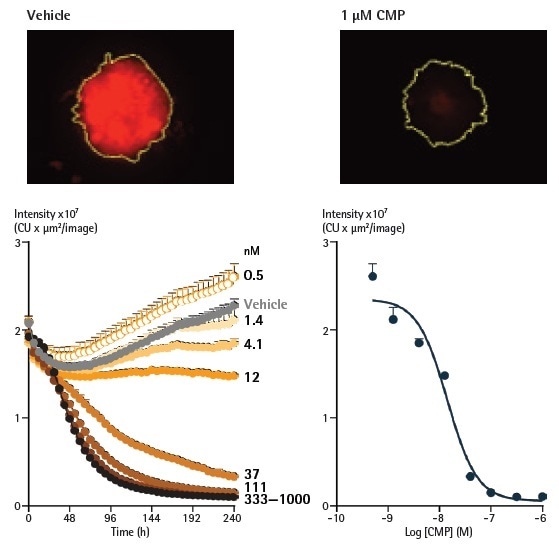
Figure 6. Analysis of spheroids expressing fluorescent proteins enables spheroid viability determination. Representative images taken at 240 h show a strong red fluorescent signal in a vehicle control spheroid, in contrast to a marked loss in red fluorescence in the CMP-treated spheroid. The yellow boundary in the images represents the Brightfield mask outline. Monitoring the integrated intensity from within the Brightfield boundary highlights a gradual increase in fluorescence under vehicle control conditions (grey symbols) corresponding to the growth of the spheroid. Upon treatment with CMP, a concentration-dependent reduction in integrated fluorescence is observed, with abolishment of fluorescence with the highest concentration tested after 240 h. Image Credit: Sartorius
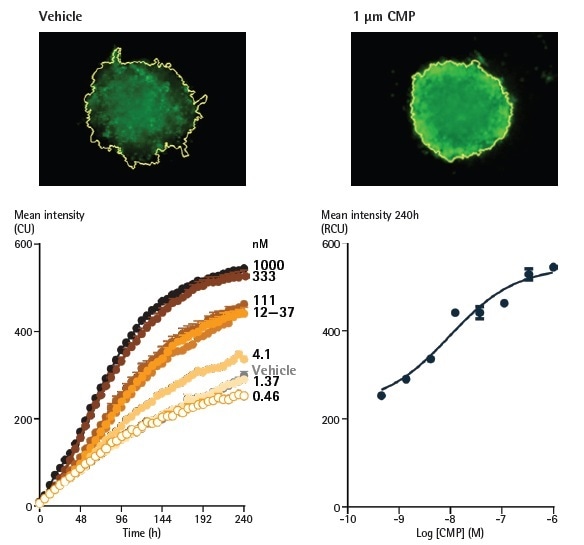
Figure 7. Effect of camptothecin (CMP) on A549 cells reported by Annexin V Green reagents in a 3D spheroid assay. A549 cells were seeded at a density of 2,500 cells/well in ULA round bottom plates and spheroids were formed for 96 h. Spheroids were treated with CMP (0.5 nM - 1 µM) or vehicle (0.1% DMSO), and apoptosis was reported using Annexin V Green. Image Credit: Sartorius
Lastly, both cell health and Brightfield readouts may be used together in the single spheroid assay to establish the drug function mechanism.
While it is effective as no labeling is required, the Brightfield analysis does not simply differentiate between cytostatic and cytotoxic agents because of the powerful Brightfield signature of nonviable spheroids.
Opposingly, establishing spheroid viability has the potential to distinguish between cytostatic and cytotoxic agents, with the former predicted to generate significant effects on the size of spheroids while promoting minimum cytotoxicity.
To show the pharmacology versatility and applicability, the influences of the cytostatic agent cycloheximide and the cytotoxic agent camptothecin were assessed on SKOV3 spheroids produced in the presence of the cytotoxicity marker Incucyte® Cytotox Green (Figure 8).
Each of the agents yielded concentration-dependent attenuation of spheroid growth, producing IC50 values of 1.5 µM for CHX and 24.6 nM for CMP. CMP interestingly induced a significant increase in the mean fluorescence, indicating a cytotoxic mechanism.
The CMP EC50 value for cytotoxicity was 51.4 nM, equivalent to the figure identified for the Brightfield (size) measurement. Opposingly, CHX only induced a significant increase in fluorescence at the largest concentration measured (10 µM), not enabling an EC50 to be established.
The distinct separation between the cytotoxicity and size readouts assists the cytostatic function of CHX.
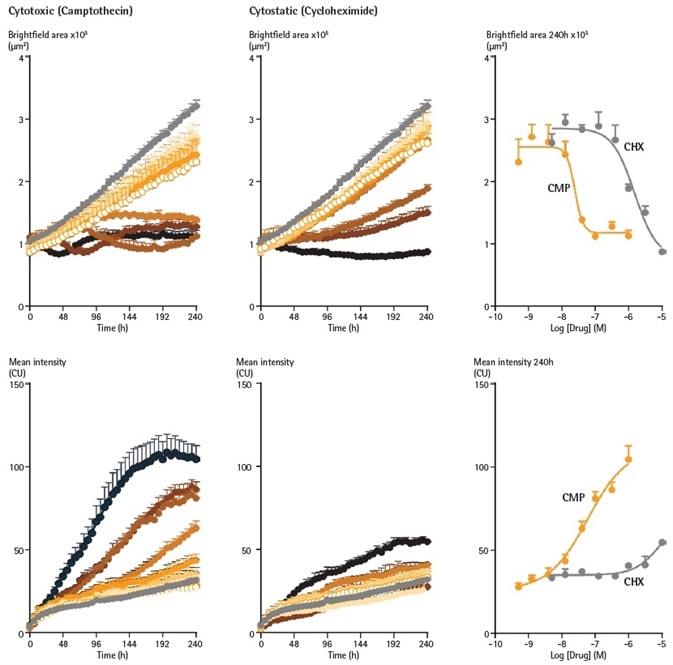
Figure 8. Cytotoxic and cytostatic mechanisms of action can be differentiated by measuring spheroid size and viability. SKOV3 cells were plated at a density of 2,500 cells per well and spheroid allowed to form (96 h). Spheroids were treated with increasing concentrations of camptothecin (0.5 nM – 1 µM) or cycloheximide (1.4 nM – 10 µM) in the presence of Cytotox Green reagent (25 nM). Images were taken every 6 h for 10 days. Time courses show change in Brightfield area (top row) or fluorescent response (bottom row) over time. CRCs show the different profiles of cytotoxic and cytostatic mechanisms. Image Credit: Sartorius
Conclusions
In this white paper, the Incucyte® Live-Cell Analysis system has been shown to assist in the investigation of 3D single spheroid analysis in real time, and that this application can be highly pragmatic for scientific research along with downstream pharmacological investigation.
Brightfield, along with phase contrast imaging, enables the label free investigation of 3D spheroid growth, shrinkage, and morphology in 96- and 384-assay formats.
Incucyte® HD phase images provide detailed visualization of spheroid morphological characteristics (size, shape) and intercellular compaction (compact aggregates vs. loose spheroids) known for each type of cell.
BF gives a method for objective spheroid kinetic measurement and cell dependent growth rate profile evaluation for a wide range of spheroids.
This platform’s kinetic analyses, along with fluorescent probes or Incucyte® Cell Health Reagents, were shown to allow the investigation of spheroid viability in real time.
The combination of viability determination with the known advantages of phase contrast morphological information and of Brightfield size analysis, produces a detailed spheroid analysis solution in one simple platform.
With no requirement for predetermined end-point selection, a highly reproducible well-to-well kinetic data, robust intra- and inter-plate reproducibility, and consistent Brightfield segmentation, the Incucyte® shows its relevance to pharmaceutical research.
The simple to use platform allows users to evaluate real time data, conduct analysis, and produce publication ready results, efficiently and quickly.
The use of the Incucyte® S3 Spheroid Software module, the Incucyte® reagents, and the Incucyte® S3 Single Spheroid Assays delivers an exclusive workflow niche, in the form of real time kinetic data, to guide both primary science research along with drug development and discovery.
References and further reading
1. Elliott NT and F Yuan. A Review of three-dimensional in vitro tissue models for drug discovery and transport studies. Pharmaceutical Science; 100:59-74 (2011)
2. Costa EC et al. 3D tumor spheroids: an overview on the tools and techniques used for their analysis. Biotechnol Adv., Dec; 34(8);1427-1441 (2016)
3. Mehta G et al. Opportunities and challenges for use of tumor spheroids as models to test drug delivery and efficacy. J. Control Release, 164; 192–204. (2012)
Discover more information on the Publications and Webinars pages.
Further information is available online from www.essenbioscience.com
Incucyte® S3 Single Spheroid Assays
Incucyte® S3 Spheroid Software Module
Watch Videos of Real Time Kinetic Analysis in the Incubator
Related protocols
- Incucyte® S3 Spheroid Viability Assay- Cell Health Reagent
- Incucyte® S3 Spheroid Viability Assay- Fluorescent Label
- Incucyte® S3 Immune Cell Killing of Tumor Spheroids
Related applications
- Incucyte® Proliferation Assays for Live-Cell Analysis
- Cytotoxicity Assays for Live-Cell Analysis
- Apoptosis Assays for Live-Cell Analysis
- Incucyte® Immune Cell Killing Assays for Live-Cell Analysis
Product inquiry
Online Product Inquiry
Acknowledgments
Produced from materials originally authored by Gillian Lovell1, Susana Alcantara1, Miniver Oliver1, Kalpana Patel1, Jill Granger2, Nevine Holtz2, Eric Endsley2, Tim Dale1, and Del Trezise1 from:
1. Essen Bioscience Ltd., United Kingdom
2. Essen BioScience Inc., USA
About Sartorius
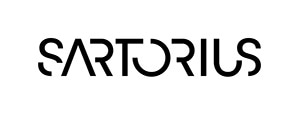
Sartorius is a leading international pharmaceutical and laboratory equipment supplier. With our innovative products and services, we are helping our customers across the entire globe to implement their complex and quality-critical biomanufacturing and laboratory processes reliably and economically.
The Group companies are united under the roof of Sartorius AG, which is listed on the Frankfurt Stock Exchange and holds the majority stake in Sartorius Stedim Biotech S.A. Quoted on the Paris Stock Exchange, this subgroup is comprised mainly of the Bioprocess Solutions Division.
Innovative Technologies Enable Medical Progress
A growing number of medications are biopharmaceuticals. These are produced using living cells in complex, lengthy and expensive procedures. The Bioprocess Solutions Division provides the essential products and technologies to accomplish this.
In fact, Sartorius has been pioneering and setting the standards for single-use products that are currently used throughout all biopharmaceutical manufacturing processes.
Making Lab Life Easier
Lab work is complex and demanding: Despite repetitive analytical routines, lab staff must perform each step in a highly concentrated and careful way for accurate results.
The Lab Products and Services Division helps lab personnel excel because its products, such as laboratory balances, pipettes and lab consumables, minimize human error, simplify workflows and reduce physical workloads
Sponsored Content Policy: News-Medical.net publishes articles and related content that may be derived from sources where we have existing commercial relationships, provided such content adds value to the core editorial ethos of News-Medical.Net which is to educate and inform site visitors interested in medical research, science, medical devices and treatments.