Due to significant developments in neuroscience techniques, researchers are now able to selectively exploit neural systems in conscious animals, through emerging methods called chemogenetics and optogenetics. These methods help in exploring the neural circuitry underlying intricate behaviors in disease and health.
Chemogenetics and optogenetics show similarities in their approach of modifying neuronal activity; for example, in both techniques, ion channels or engineered receptors have to be introduced in particular brain regions, through plasmid expression or viral vector systems. In optogenetics, bacterial light-sensitive ion channels must be expressed and fiber optics also have to be subsequently used to inhibit or activate neuronal activity in vivo or in vitro (Boyden et al., 2005; Zhang et al., 2007).
Although this method offers superior temporal control of in vivo neuronal activity, it is known to be inherently invasive and needs cerebral implantation of fiber optics. Chemogenetics, on the other hand, does not need a chronic implant but maintains the potential to control neuronal activity. This is accomplished by administering ligands, selective for ion channels or engineered receptors, which are otherwise inert (Armbruster et al., 2007; Campbell & Marchant, 2018). Table 1 shows the main features of chemogenetics and optogenetics.
Table 1. Chemogenetics vs optogenetics
|
Chemogenetics |
Optogenetics |
Method of intervention |
Inert, small molecule ligands selective for genetically engineered receptors/ion channels |
Light-sensitive ion channels activated by implanted fiber optics |
Is the intervention ‘physiological’? |
Yes – uses conserved, intracellular signaling pathways, or changes in ion channel conductance, to alter neuronal activity |
No – patterns of excitation/inhibition are artificially synchronized by light stimulation pattern |
Is the intervention inert? |
Yes – receptors/ion channels lack pharmacological activity without ligands and ligands are pharmacologically inert without specific receptors/ion channels |
No – the fiber optic light source can create heat and bacterial light-sensitive channels used can be antigenic |
Is this method invasive? |
Minimally to no – ligands can be given by intracerebral infusion, intraperitoneal injection or in drinking water, dependent on specific ligand |
Yes – inherently invasive due to implantation of fiber optics |
Is specialized equipment required? |
No |
Yes – requires implantable fiber optics as a light source |
History and Development
RASSLs
Chemogenetics refers to the use of ion channels or genetically engineered receptors and the selective ligands activating those receptors to facilitate the manipulation of neuronal activity. In this context, G-protein coupled receptors (GPCRs) have been spearheading the development of chemogenetics, and the original paper defining GPCRs that react only to synthetic ligands was published in 1998.
These receptors — known as Receptors Activated Solely by a Synthetic Ligand (RASSLs) — were effectively applied in vivo, for instance, to remotely control cardiac activity. Despite this fact, the application of RASSLs in neuroscience has been limited by the endogenous activity of receptors in the absence of their particular ligand and the pharmacological activity of ligands in vivo (Coward et al., 1998; Sternson & Roth, 2014).
DREADDs
The recent years have seen the development of Designer Receptors Exclusively Activated by Designer Drugs (DREADDS). Mutated human muscarinic receptors stimulated only by inert ligands were the first DREADDS to be developed (Armbruster et al., 2007). Through several rounds of mutagenesis and screening against the biologically inert ligand Clozapine N-oxide (CNO), muscarinic receptors coupled to the Gαq intracellular signaling pathway were identified.
Receptors coupled to this pathway are capable of activating neuronal activity in response to CNO. Low concentrations of CNO activate all three Gαq-DREADDs — hM1Dq, hM3Dq, and hM5Dq (Roth, 2016). Moreover, the same study revealed that hM4Di and hM2Di can inhibit neuronal activity via their coupling to Gαi intracellular signaling pathways. These inhibitory DREADDs also respond to CNO (Armbruster et al., 2007; Figure 1).
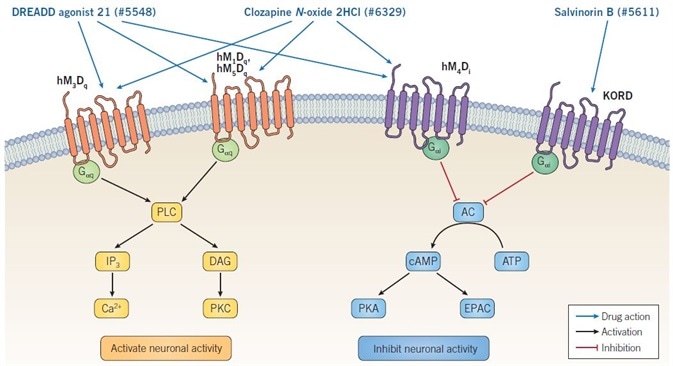
Figure 1. Mechanism of action of DREADD ligands. Binding of DREADD ligands to Gαq-DREADDs provokes neuronal firing, whereas binding to Gαi-DREADDs results in inhibition of neuronal activity. Clozapine N-oxide dihydrochloride and DREADD agonist 21 are non-selective muscarinic DREADD agonists and so can activate or inhibit neuronal activity, depending on the specific receptor being expressed. Salvinorin B is selective for the KORD receptor, which is coupled to GαI signaling, consequently binding results in inhibition of neuronal activity. Image credit: Tocris Bioscience
The binding between Gαq-DREADDs and CNO causes the stimulation of phospholipase C (PLC), which catalyzes the conversion of phosphatidylinositol 4,5-bisphosphate (PIP2) to 1,2-diacylglycerol (DAG) and inositol 1,4,5-trisphosphate (IP3). Both DAG and IP3 possess second messenger functions: the latter binds to its receptors to trigger the release of Ca2+ from intracellular stores, whereas the former stimulates various forms of protein kinase C (PKC).
The binding between Gαi-DREADDs and CNO causes the inhibition of adenylyl cyclase (AC), resulting in decreased intracellular cAMP levels. Since both EPAC and protein kinase A (PKA) are activated by cAMP, the CNO’s action at Gαi-DREADDs inhibits EPAC and PKA downstream signaling (see Figure 1).
CNO is a metabolite of clozapine, studies indicate that this is a bidirectional conversion and that CNO can undergo reverse metabolism to clozapine. When CNO is administered at the concentrations needed to activate DREADDs, clozapine is subsequently able to activate endogenous receptors (Gomez et al., 2017). Clozapine — an atypical antipsychotic — acts at an array of targets and leads to many different behavioral effects.
Rats, mice, humans, non-human primates, and guinea pigs all show the reversible metabolism of CNO to clozapine (Gomez et al., 2017; Manvich et al., 2018). As a result of potential reverse metabolism of CNO, structure-activity relationship studies have evolved to develop stable, alternative ligands.
The potent DREADD ligand — DREADD agonist 21 — was initially analyzed for activity against hM3Dq. The approved drug perlapine was identified as a powerful hM3Dq agonist in the same research. In Japan, this drug has been approved as a sedative and hypnotic (Chen et al., 2015). Both perlapine and DREADD agonist 21 have been subsequently shown to be potent agonists of hM4Di, hM3Dq, and hM1Dq with little to no off-target activity. Moreover, DREADD agonist 21 has been tested in vivo, in which it has been demonstrated to activate hM3Dq-expressing neurons and inhibit the activity of hM4Di-expressing neurons (Thompson et al., 2018).
Subsequent to the development of muscarinic DREADDs, an inhibitory DREADD has been created from the κ-opioid receptor (KORD). Activation of this inhibitory DREADD is achieved through binding of the ligand Salvinorin B, resulting in the inhibition of neuronal activity through Gαi signaling. To allow bidirectional control of neuronal activity, KORD can be utilized alongside, activating DREADDs like hM3Dq (Vardy et al., 2015).
Some common features in all DREADDs make them suitable for application in neuroscience experiments. Firstly, DREADDs do not exhibit any response to endogenous ligands because of genetic mutations within their ligand binding sites that eliminate binding, implying that any activity of the DREADD will be only because of the applications of the specific DREADD ligand. Secondly, in vitro or in vivo expression of DREADDs does not have any impact on baseline behaviors, neuronal function, or cellular activity, before the addition of the DREADD ligand (Sternson & Roth, 2014).
PSAMs/PSEMs
While DREADDs and RASSLs are based on GPCRs, modified ion channels named Pharmacologically Selective Actuator Modules (PSAMs), have also been used for modulating the activity of neurons. PSAMs are based on studies indicating that it is possible to transplant the extracellular ligand binding domain of the α7 nicotinic ACh receptor (nAChR) onto the ion pore domain of other ligand-gated ion channels. When the α7 nAChR ligand binding domain is spliced with the ion pore domain of the 5-HT3 receptor, an ion channel with α7 nAChR pharmacology is produced but with 5-HT3 cation conduction properties (Eiselé et al., 1993).
Likewise, splicing of the α7 nAChR ligand binding domain with the ion pore domain of the chloride selective glycine receptor (GlyR) produces an ACh responsive chloride channel (Grutter et al., 2005). Selective mutation of the α7 nAChR ligand binding domain consequently generates PSAM ion channels, which do not show any ACh binding, but are still selectively bound by compounds called Pharmacologically Selective Effector Molecules (PSEMs).
PSAMs or chimeric ion channels allowing regulation of anion or cation conductance have been produced through the combination of the mutated α7 nAChR ligand binding domain (harboring two or one mutations) with the ion pore domain of several varied ligand-gated ion channels. Such PSAM chimeras are named based on their mutations as well as linked ion pore domain — PSAML141F,Y115F-GlyR, PSAML141F-GlyR, PSAML141F,Y115F-GABAC, and PSAML141F,Y115F-5-HT3. Activation of neuronal activity is enabled by 5-HT3-containing chimeras, whereas GABAC- and GlyR-containing chimeras are inhibitory (see Figure 2) (Magnus et al., 2011; Sternson & Roth, 2014).
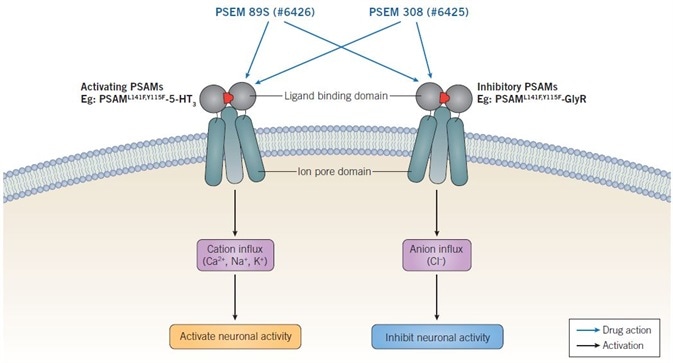
Figure 2. Mechanism of action of PSEMs. Activating PSAMs are composed of a mutated α7 nAChR ligand binding domain spliced with the ion pore domain of a cation-selective channel, such as 5-HT3. Binding of PSEMs to activating PSAMs results in an influx of cations and activation of neuronal activity. Inhibitory PSAMs are composed of a mutated α7 nAChR ligand binding domain spliced with the ion poredomain of an anion selective channel, such as GlyR. Binding of PSEMs to inhibitory PSAMs results in an influx of anions and inhibition of neuronal activity. Image credit: Tocris Bioscience
Scientific reviews for further reading
- Campbell & Marchant (2018) The use of chemogenetics in behavioral neuroscience: receptor variants, targeting approaches and caveats. Br J Pharmacol. 175, 994.
- Roth (2016) DREADDs for Neuroscientists. Neuron. 89, 683.
- Magnus et al. (2011) Chemical and genetic engineering of selective ligand-ion channel interactions. Science. 333, 1292.
- Sternson & Roth (2014) Chemogenetic tools to interrogate brain functions. Annu Rev Neurol. 37, 387.
References
- Armbruster et al. (2007) Evolving the lock to fit the key to create a family of G protein-coupled receptors potently activated by an inert ligand. Proc Natl Acad Sci USA. 104, 5163.
- Atasoy et al. (2012) Deconstruction of a neural circuit for hunger. Nature. 488, 172.
- Boyden et al. (2005) Millisecond-timescale, genetically targeted optical control of neural activity. Nat Neurosci. 8, 1263.
- Bradley & Tobin (2016) Design of next-generation G protein-coupled receptor drugs: linking novel pharmacology and in vivo animal models. Annu Rev Pharmacol Toxicol. 56, 535.
- Bradley et al. (2018) The use of chemogenetics approaches to study the physiological roles of muscarinic acetylcholine receptors in the central nervous system. Neuropharmacology. 136, 421.
- Chen et al. (2015) The first structure-activity relationship studies for designer receptors exclusively activated by designer drugs. ACS Chem Neuroscience. 6, 476.
- Coward et al. (1998) Controlling signaling with a specifically designed Gi-coupled receptor. Proc Natl Acad Sci USA. 95, 352.
- Eiselé et al. (1993) Chimaeric nicotinic-serotonergic receptor combines ligand binding and channel specificities. Nature. 366, 479.
- Ge et al. (2017) Glutamatergic projections from the entorhinal cortex to dorsal dentate gyrus mediated context-induced reinstatement of heroin seeking. Neuropsychopharmacology. 42, 1860.
- Gomez et al. (2017) Chemogenetics revealed: DREADD occupancy and activation via converted clozapine. Science. 357, 503.
- Grutter et al. (2005) Molecular tuning of fast gating in pentameric ligand-gated ion channels. Proc Natl Acad Sci USA. 102, 18207.
- Jiang et al. (2018) Cholinergic neurons in medial septum maintain anxiety-like behaviors induced by chronic inflammatory pain. Neurosci Lett. 671, 7.
- Manvich et al. (2018) The DREADD agonist clozapine N-oxide (CNO) is reverse metabolized to clozapine and produces clozapine-like interoceptive stimulus effects in rats and mice. Sci Rep. 8, 3840.
- Rapanelli et al. (2017) Histamine modulation of the basal ganglia circuitry in the development of pathological grooming. Proc Natl Acad Sci USA. 114, 6599.
- Sasaki et al. (2011) Pharmacogenetic modulation of orexin neurons alters sleep/wakefulness states in mice. PLoS One. 6, e20360.
- Schwartz et al. (2017) Cortico-accumbens regulation of approach-avoidance behavior is modified by experience and chronic pain. Cell Rep. 19, 1522.
- Thompson et al. (2018) DREADD agonist 21 (C21) is an effective agonist for muscarinic-based DREADDs in vitro and in vivo. ACS Pharmacol Transl Sci. Epub ahead of print.
- Vardy et al. (2016) A new DREADD facilitates the multiplex chemogenetic interrogation of behavior. Neuron. 86, 936.
- Varela et al. (2016) Tracking the time-dependent role of the hippocampus in memory recall using DREADDs. PLoS One. 11, e0154374.
- Zhang et al. (2007) Multimodal fast optical interrogation of neural circuitry. Nature. 446, 633.
About Tocris Bioscience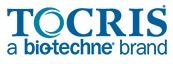
Tocris Bioscience is your trusted supplier of high-performance life science reagents, including receptor agonists & antagonists, enzyme inhibitors, ion channel modulators, fluorescent probes & dyes, and compound libraries. Our catalog consists of over 4,500 research tools, covering over 400 protein targets enabling you to investigate and modulate the activity of numerous signaling pathways and physiological processes.
We have been working with scientists for over 30 years to provide the life science community with research standards, as well as novel and innovative research tools. We understand the need for researchers to trust their research reagents, which is why we are committed to supplying our customers with the highest quality products available, so you can publish with confidence.
Tocris is part of the protein sciences division of Bio-Techne, which also includes the best in class brands R&D Systems, Novus Biologicals, ProteinSimple, and Advanced Cell Diagnostics. Bio-Techne has united these brands to provide researchers with a full portfolio of research reagents, assays, and protein platforms. For more information on Bio-Techne and its brands, please visit bio-techne.com.
Sponsored Content Policy: News-Medical.net publishes articles and related content that may be derived from sources where we have existing commercial relationships, provided such content adds value to the core editorial ethos of News-Medical.Net which is to educate and inform site visitors interested in medical research, science, medical devices and treatments.