The Endocannabinoid System
Cannabinoid receptors that have been identified so far are of two types1,2: the CB1 receptor cloned in 19903 and the CB2 receptor cloned in 19934. Both receptors belong to the superfamily of G-protein-coupled receptors (GPCRs). The cloning of these receptors was the motivation for developing mice in which cannabinoid CB1 and/or CB2 receptors have been deleted genetically. Currently, these transgenic animals, specifically CB1 knockout mice, are extensively used to investigate the pathological and physiological functions of cannabinoid receptors1,5,6.
CB1 receptors are predominantly located at the terminals of peripheral and central neurons where they often mediate inhibition of the release of neurotransmitters. They also exist in certain non-neuronal cells, such as immune cells. CB2 receptors are mainly found in immune cells, both inside and outside the central nervous system. These receptors perform functions within immune cell migration and modulation cytokine release. In the brain, these receptors are expressed by blood vessels and microglia,7 and by certain neurons8,9. Yet, researchers are still unaware of the role played by neuronal CB2 receptors.
CB1 receptors’ central distribution pattern is heterogeneous and accounts for various notable pharmacological properties of CB1 agonists, for instance, their potential to affect memory and cognition as well as to modify the control of motor function. Hence, specifically high concentrations of CB1 receptors are found in the hippocampus, cerebral cortex, substantia nigra pars reticulata, lateral caudateputamen, entopeduncular nucleus, globus pallidus, and the molecular layer of the cerebellum1,10. In accordance with the analgesic properties of cannabinoid receptor agonists, CB1 receptors are also found on pain pathways in the spinal cord and brain as well as at the peripheral terminals of primary sensory neurons11,12.
Despite the fact that the population of CB1 receptors is significantly less in peripheral tissues compared to their concentration in the central nervous system, this does not imply that peripheral CB1 receptors are insignificant. In certain peripheral tissues, discrete regions. like nerve terminals that constitute only a trivial portion of the total tissue mass, are known to possess high concentrations of CB1 receptors. CB1 receptors are also expressed on neurons in peripheral tissues such as the urinary bladder, vas deferens, the heart, and small intestine10,13.
Gi/o proteins couple to both CB1 and CB2 receptors, positively modulating mitogen-activated protein kinase (MAPK) and negatively modulating adenylyl cyclase (AC)1,14. They also couple CB1 receptors to ion channels: negatively to CaV2.2 (N-type) and CaV2.1 (P/Q-type) calcium channels and positively to A-type voltage-gated (KV), and inwardly rectifying (Kir) potassium channels1,13,14.
In addition, CB1 receptors can activate adenylyl cyclase by coupling to Gs proteins15–17. The extent to which this takes place is probably governed by where these receptors are located or by cross-talk between CB1 receptors and co-localized G-protein-coupled non-CB1 receptors15,16,18,19. There is a likelihood that CB1 receptors occur as two distinct subpopulations, one of which is coupled to Gi/o proteins and the other coupled to Gs proteins15. Additional signaling mechanisms for cannabinoid CB1 and CB2 receptors have also been proposed elsewhere1,14.
After cannabinoid receptors were cloned, it was discovered that mammalian tissues synthesize compounds with the potential to activate these receptors. N-arachidonoyl ethanolamine (anandamide) identified in 1992 and 2-arachidonylglycerol identified in 1995 were the first-ever endogenous cannabinoids (endocannabinoids) identified20–22. Both of these are produced on demand as a reaction to an increase in intracellular calcium23. N-acyl phosphatidylethanolamine-selective phospholipase D (NAPE-PLD) catalyzes the process in which anandamide is produced from N-arachidonoyl phosphatidylethanolamine. However, it is thought that the production of 2-arachidonylglycerol is dependent on the transformation of 2-arachidonate-containing phosphoinositides to diacylglycerols, and on their subsequent conversion to 2-arachidonylglycerol by DAGLα and DAGLβ, two diacylglycerol lipase (DAGL) isozymes23,24.
Once these endocannabinoids are produced and released, cellular uptake removes them from their sites of action and they are degraded by enzymes; 2-arachidonylglycerol mainly by monoacylglycerol lipase (MAGL) but also by fatty acid amide hydrolase (FAAH), and anandamide by FAAH and/or by palmitoylethanolamide-preferring acid amidase (PAA), cyclooxygenase-2, lipoxygenases, and cytochrome P4505,23–25.
O-arachidonoyl ethanolamine (virodhamine), 2-arachidonylglyceryl ether (noladin ether), N-docosatetraenoyl ethanolamine, N-dihomo-γ-linolenoyl ethanolamine, N-arachidonoyl dopamine (NADA), oleamide, and N-oleoyl dopamine (OLDA) are other ligands that could be endocannabinoids5. Along with their receptors, endocannabinoids form what is now termed the “endocannabinoid system”.
Although it is usually agreed that endocannabinoids pass through cell membranes, one problem that is now a matter of dispute is whether a transporter mediates the cellular uptake of endocannabinoids, like anandamide25–27. On the other hand, currently, FAAH is well characterized. As a matter of fact, it has been cloned28 and FAAH knockout mice have been developed29,30. In addition, NAPE/PLD31, MAGL32–34, and DAGLα and DAGLβ35 have been cloned and mice with a genetic deletion of NAPE/PLD have been developed36.
It has been found that at least some of the effects brought about by endogenously released anandamide and 2-arachidonylglycerol seem to be elevated by the so-called “entourage effect.” This is dependent on the co-release of other endogenous fatty acid derivatives, including oleamide and palmitoylethanolamide, which can potentiate anandamide, and 2-palmitoylglycerol and 2-linoleoylglycerol, which can potentiate 2-arachidonylglycerol37. The mechanism(s) that cause the entourage effect have not yet been determined.
It is most likely that endocannabinoids have immunomodulatory as well as neuromodulatory roles, including the suppression of ongoing transmitter release via retrograde signaling38 and the regulation of cytokine release and immune cell migration39,40. Moreover, it is also widely acknowledged that in some disorders there is an increase in endocannabinoid release in specific tissues, and furthermore, in certain cases this upregulation of the endocannabinoid system results in the inhibition of unwanted signs and symptoms and so is “autoprotective”, while in others it leads to undesirable effects5. Hence, there is evidence that the release of endocannabinoids in one way ameliorates spasticity in inflammatory pain and multiple sclerosis, and in another way it leads to obesity in certain individuals or affects fertility in some women. Consequently, at present, there is huge interest not just in cannabinoid receptor agonists and antagonists that act directly but also in compounds that can have an indirect effect on the activity of the endocannabinoid system through allosteric modulation of endocannabinoid-induced activation of cannabinoid receptors, or by modifying the concentration of endocannabinoids at their receptors by means of effects on endocannabinoid production or degradation.
The rest of this article deals with the main pharmacological actions of several such direct and indirect cannabinoid receptor agonists and antagonists. The focus is specifically on those compounds that are most extensively used as experimental tools in cannabinoid research. Where possible, citations to previous review articles have been included that offer more detailed information.
Mixed CB1/CB2 Receptor Agonists
In earlier studies1,2,41, it has been reported that compounds known to activate CB1 and CB2 receptors with almost equal potency are most often used in the lab as CB1/CB2 receptor agonists, and typically fall into one of four chemical categories: aminoalkylindole, classical cannabinoid, nonclassical cannabinoid, and eicosanoid. Several CB1/CB2 receptor agonists that are extensively used consist of chiral centers and normally present signs of stereoselectivity in pharmacological assays where the measured response is CB1 or CB2 receptor-mediated1,2,41. In general, (–)-trans (6aR, 10aR) classical and nonclassical cannabinoids present considerably greater potency as cannabinoid receptor agonists when compared to their (+)-cis (6aS, 10aS) enantiomers. Three prominent examples of such compounds are (–)-Δ9-tetrahydrocannabinol (Δ9-THC), (–)-11-hydroxy-Δ8-THC-dimethylheptyl (HU 210), and CP 55,940.
With regards to WIN 55,212, an aminoalkylindole, its (R)-(+)-isomer (WIN 55,212-2) presents considerable agonist activity at both CB1 and CB2 receptors, but its (S)-(–)-isomer (WIN 55,212-3) does not. In fact, upon administering WIN 55,212-3 in vitro at concentrations in the low micromolar range, it has been observed to act as a partial inverse agonist at CB1 receptors and as a neutral CB2 receptor antagonist44. Anandamide, an eicosanoid cannabinoid, does not containg any chiral centers. Yet, some of its synthetic equivalents—for example, the CB1-selective agonist methanandamide (see next section)—do contain chiral centers. The (R)-(+)-isomer of methanandamide exhibits nine times increased affinity for CB1 receptors when compared to the (S)-(–)-isomer45.
The low water solubility and high lipophilicity of the majority of CB1 and CB2 receptor ligands are major practical challenges within cannabinoid research, both in vivo and in vitro, as this demands the use of a non-aqueous vehicle like polyvinylpyrrolidone, ethanol, Emulphor, Cremophor, dimethyl sulfoxide, Tween 80, bovine serum albumin, or the water-soluble emulsion Tocrisolve 100, which is a blend of Pluronic F68, soya oil, and water1,46,47. As a result, the Organix compound 3-(5′-cyano- 1′,1′-dimethylpentyl)-1-(4-N-morpholinobutyryloxy)-Δ8-THC hydrochloride (O-1057)48 is one other cannabinoid CB1/CB2 receptor agonist that deserves special reference as it is readily water-soluble. O-1057’s in vitro potency when compared to that of CP 55,940 is just 2.9 times less at CB1 receptors and 6.5 times less at CB2 receptors.
CB1-Selective Agonists
The anandamide molecule was the starting point for the development of the first CB1-selective agonists. The marginal CB1 selectivity of this molecule can be considerably improved by introducing a fluorine atom on the terminal 2′ carbon to produce O-585 and/or by substituting a methyl group in the place of a hydrogen atom on the 1′ or 2 carbon to produce (R)-(+)-methanandamide, its cyano equivalent O-1812, or O-6891,2,41. Another significant outcome of introducing a methyl group on the 1′ or 2 carbon is enhanced resilience to the hydrolytic action of FAAH. In fact, (R)-(+)-methanandamide was first produced in Dr Alexandros Makriyannis’ lab to satisfy the need for an anandamide equivalent that is metabolically more stable. Apart from O-181249, arachidonyl-2′-chloroethylamide (ACEA) and arachidonylcyclopropylamide (ACPA) have been the most potent CB1-selective agonists developed so far. Both of these present fairly high CB1 efficacy.50
In contrast to O-1812, or in fact O-689 or methanandamide, neither ACEA nor ACPA exhibit any resilience to enzymic hydrolysis.1,2,49 This is possibly due to the fact that they lack a methyl substituent on the 1′ or 2 carbon and, actually, it has been demonstrated that the introduction of a methyl group to the 1′ carbon of ACEA evidently reduces the vulnerability of this molecule to FAAH-mediated hydrolysis51. In addition, this structural change decreases ACEA’s affinity for CB1 receptors by nearly 14 times. 2-arachidonylglyceryl ether (noladin ether) is one more arachidonic acid derivative that is worth mentioning as a CB1-selective agonist, more so as it is a putative endocannabinoid52. When compared to CP 55,940, this ligand presents CP 55,940-like CB1 efficacy yet less CB1 potency53,54.
CB2-Selective Agonists
JWH 133, the classical cannabinoid, and JWH 015, the less selective aminoalkylindole have been the CB2-selective agonists most extensively used as experimental tools. Both of these were synthesized by Dr John Huffman1,2,72. Aside from more readily binding to CB2 than to CB1 receptors, each of these agents also acts as a potent CB2-selective agonist in functional assays. GW 405833, the GlaxoSmithKline compound that acts as a potent partial agonist at the CB2 receptor67, as well as AM 1241, HU 308, and the Merck Frosst compounds L-759,633 and L-759,656 are other prominent CB2-selective agonists1,2. Fascinatingly, AM 1241 could be a “protean agonist” since it has been found to act as an agonist in tissues naturally expressing CB2 receptors, but not in tissues where CB2 receptors are genetically inserted and are hence possibly overexpressed73.
Selective CB1 Receptor Antagonists/Inverse Agonists
SR141716A, the diarylpyrazole, was the first one to be developed among these56. This selective and highly potent CB1 receptor ligand readily blocks or reverses in vitro and in vivo CB1-mediated effects1,2,41. AM 251, AM 281, and LY 320135 are other notable CB1-selective antagonists. Both AM 251 and AM 281 were developed by Dr Alexandros Makriyannis. When compared to AM 251, AM 281, or SR141716A, LY 320135 has less affinity for CB1 receptors and at in the low micromolar range concentrations, it also binds to muscarinic and 5-hydroxytryptamine (5-HT2) receptors1,2,41.
As described in other studies,2,74 there is evidence that AM 251, AM 281, LY 320135, and SR141716A are not “neutral” antagonists. Hence, in addition to mitigating the effects of CB1 receptor agonists, they can by elicit responses on their own in certain CB1 receptor-containing tissues that are in a direction opposite from those elicited by CB1 receptor agonists. Although in some cases “inverse cannabimimetic effects” such as these might be due to a direct antagonism of responses evoked at CB1 receptors by released endocannabinoids, there is research indicating that this is not always the underlying mechanism, and that AM 251, AM 281, LY 320135, and SR141716A are actually inverse agonists74. In particular, they seem to generate inverse cannabimimetic effects in certain tissues by reducing the constitutive activity of CB1 receptors in some way (the coupling of CB1 receptors to their effector mechanisms that, it is considered, can take place without endogenously released or exogenously added CB1 agonists).
Selective CB2 Receptor Antagonists/ Inverse Agonists
SR14452869, the Sanofi-Aventis diarylpyrazole, and 6-iodopravadoline (AM 630)71 are the most prominent CB2-selective antagonists/inverse agonists. Both the compounds bind to CB2 with considerably higher affinity compared to CB1 receptors, present pronounced potency similar to CB2 receptor antagonists, and act as inverse agonists that have the ability to bring about inverse cannabimimetic effects at CB2 receptors on their own1,2,41. It has been reported that AM 630 reverses CP 55,940-induced suppression of the production of forskolin-stimulated cyclic AMP by human CB2-transfected CHO cell preparations at nanomolar concentrations (EC50 = 129 nM). It has also been observed to increase the production of forskolin-stimulated cyclic AMP by the same cell line upon being administered by itself (EC50 = 230 nM)71, though with efficacy that seems to be slightly less than the inverse efficacy exhibited by SR144528 in this bioassay75. In certain tests, AM 630 has been observed to act as a low-potency partial agonist41,71,76–78 at the CB1 receptor; however, in others it acts as a low-potency inverse agonist79,80.
Neutral Cannabinoid Receptor Antagonists
At present, there is much attention toward the possibility of creating potent neutral CB1 and CB2 receptor antagonists—that is, high-affinity ligands for CB1 or CB2 receptors that are deficient in essential agonist or inverse agonist efficacy. One reason for this is that, in contrast to the CB1- and CB2-selective antagonists/inverse agonists currently available (see earlier sections), it is possible to use a neutral antagonist to differentiate between tonic cannabimimetic activity that occurs due to ongoing release of endocannabinoid onto CB1 or CB2 receptors, which it will oppose, as well as tonic activity that occurs due to the existence of intrinsically active CB1 or CB2 receptors, which it will not oppose.
Despite the fact that it has not been possible to develop neutral antagonists that selectively target the CB2 receptor, some advancement has been made with respect to the CB1 receptor. There is some evidence that 6″-azidohex-2″-yne-cannabidiol (O-2654), O-2050, a sulfonamide equivalent of Δ8-THC that has an acetylenic side chain, and VCHR, an equivalent of SR141716A, are neutral CB1 receptor antagonists2,74. Since this proof is somewhat preliminary, there should be discretion while using any of these ligands as a pharmacological tool. There exists more comprehensive proof that NESS 0327, another SR141716A equivalent, is a neutral CB1 receptor antagonist55. Yet, at present, this compound is not available commercially and hence has not been used to a large extent in research on cannabinoids.
Radiolabeled Cannabinoid Receptor Ligands
CB1-selective [3H]SR141716A (CB1 Kd = 0.19–1.24 nM) as well as [3H]CP 55,940, [3H]WIN 55,212-2, and [3H]HU 243, are the tritiated cannabinoid receptor ligands most extensively used in binding assays or for autoradiography. The latter three compounds bind almost equally well to CB1 and CB2 receptors. Standard Kd values for [3H]CP 55,940, [3H]WIN 55,212-2, and [3H]HU 243 are 0.07–4 nM, 1.9–16.2 nM, and 0.045 nM, respectively, at CB1 receptors and 0.2–7.4 nM, 2.1–3.8 nM, and 0.061 nM, respectively, at CB2 receptors2,41. [3H]HU 243—that is structurally quite similar to HU 210—has a specifically high affinity for these receptors.
In addition, radiolabeled ligands have been created as prospective probes for human positron emission tomography (PET) or single photon emission computed tomography (SPECT) experiments. These are 123I labeled equivalents of AM 251 (CB1 Kd = 0.23–0.62 nM) and AM 28181–83 and an 18F-labeled equivalent of SR141716A, i.e., SR144385.84 Notably favorable outcomes have been observed in animal experiments when [123I]AM 281 is used.82,85
Additional Pharmacological Targets for CB1 and CB2 Receptor Ligands
At present, it is widely accepted that certain cannabinoid receptor agonists are fairly potent at stimulating the TRPV1 (vanilloid VR1) receptor. These agonists include eicosanoids like ACEA, anandamide, NADA, methanandamide, and certain anandamide metabolites, as well as OLDA, the putative endocannabinoid. The list excludes 2-arachidonylglycerol as well as classical, aminoalkylindole, and nonclassical cannabinoid receptor agonists like HU 210, WIN 55,212-2, and CP 55,9402,5,88,92,93.
Several other non-CB1 and non-CB2 pharmacological targets for certain CB1/CB2 receptor agonists have been put forward, such as a number of targets that seem to respond to agonist concentrations of 1 μM or less and thus to have sensitivity to these ligands of the same order as that presented by CB1 or CB2 receptors. For instance, anandamide interacts with different types of voltage-gated and ligand-gated ion channels at submicromolar concentrations, where some (but not all) of these channels are also sensitive to Δ9-THC, 2-arachidonylglycerol, WIN 55,212-2, CP 55,940, and/or JWH 015.
There is also evidence that Δ9-THC also interacts strongly with neuronal transporters of 5-hydroxytryptamine, noradrenaline, and dopamine, and that there are several less sensitive pharmacological targets for Δ9-THC and/or for some other cannabinoid receptor agonists. These targets only appear to react to >1 μM cannabinoid concentrations and include TRPA1 and PPARγ receptors; shaker Kv1.2 K+ and L-type Ca2+ channels; putative non-TRPV1, non-CB1, and non-CB2 neuronal receptors in the small intestine; sites at gap junctions between cells; and sites on glutamate GLUA1 and GLUA3 receptors and on muscarinic M1 and M4 receptors2,86,88,94,95.
While anandamide and methanandamide also act as agonists for the putative abnormal-cannabidiol (abnormal-CBD) receptor2,88,96, WIN 55,212-2, Δ9-THC, or 2-arachidonylglycerol do not (see also section on other notable ligands). SR141716A and AM 251—the CB1 receptor antagonists/inverse agonists—also have the ability to interact with non-CB1 and non-CB2 targets, though only at concentrations in the micromolar range and thus above concentrations at which these ligands have the potential to produce CB1 receptor antagonism. Hence, for instance, as discussed in other studies74,88, it has been observed that at micromolar concentrations:
- Neuronal voltage-sensitive Na+ channels can be blocked by AM 251
- Adenosine A1 receptor activation can be blocked by AM 251 and SR141716A
- Activation of putative abnormal-CBD receptors on mesenteric arteries and of putative non-I1 and non-I2 imidazoline receptors can be blocked by SR141716A
- WIN 55,212-2-induced activation of central presynaptic putative TRPV1-like receptors can be antagonized by SR141716A and not AM 251
- Ca2+-activated (BK) K+ channels, L-type Ca2+ channels, sites at gap junctions between cells, and ATP-sensitive K+ channels can be blocked by SR141716A
It is most likely that future studies will uncover additional targets for CB1 and CB2 receptor ligands. In fact, in AstraZeneca and GlaxoSmithKline patents, it has already been claimed that certain standard cannabinoid receptor agonists, as well as antagonists, stimulate GPR55, the G-protein-coupled orphan receptor97,98.
Since cannabinoid receptor agonists vary by how much they interact with the standard or proposed non-CB1 and non-CB2 targets, it follows that certain ligands that activate CB1 and/or CB2 receptors with comparable potencies will most likely have pharmacological profiles that differ from each other. It is also worth mentioning that despite the fact that there exist several established cannabinoid receptor ligands presenting pronounced selectivity as agonists or antagonists/inverse agonists for CB1 or CB2 receptors (see earlier sections), none of these ligands are completely CB1- or CB2-specific.
Hence, it is anticipated that each of these ligands will block or activate both types of receptors equally well upon being delivered at a high enough concentration and hence will display selectivity only upon being delivered at lower concentrations or doses lying within its CB1 or CB2 “selectivity window”.
Inhibitors of 2-Arachidonylglycerol Biosynthesis
As 2-arachidonylglycerol and anandamide are produced on demand and not stored, and because there is proof for elevated production and release of either or both of these endocannabinoids to be responsible for undesirable signs and symptoms of specific disorders (see section on the endocannabinoid system), selective suppressors of their enzymic biosynthesis would not just constitute vital experimental tools but also exhibit potential as therapeutic agents.
Selective inhibitors of NAPE-PLD have not been discovered so far, but inhibitors are available for DAGLα and DAGLβ, the enzymes that catalyze the conversion of diacylglycerols to 2-arachidonylglycerol. Tetrahydrolipstatin is one such inhibitor that suppresses DAGLα (IC50 = 60 nM) and DAGLβ (IC50 = 100 nM) much more potently than it suppresses NAPE-PLD (IC50 = 10 μM). It does not suppress MAGL even at micromolar concentrations of 25 μM35,99.
O-3841 is another prominent DAGL inhibitor that suppresses DAGLα at nanomolar concentrations (IC50 = 160 nM). However, at concentrations of up to 25 μM, it lacks any noticeable inhibitory effect on FAAH, NAPE-PLD, triacylglycerol lipase, or MAGL activity or on the particular binding of [3H]CP 55,940 to human CB1 or CB2 receptors99. Although both O-3841 and tetrahydrolipstatin suppress DAGL in membrane preparations, thus far, only tetrahydrolipstatin has been observed to synthesize noticeable signs of DAGL inhibition in intact cells99.
Inhibitors of the Enzymic Hydrolysis of Endocannabinoids
The occurrence of MAGL and FAAH in various tissues has led to the need for selective inhibitors of these enzymes that can be employed to promote studies investigating the pharmacological actions of endocannabinoids upon being administered exogenously as well as their pathological and physiological roles during their endogenous release. Somewhat as a consequence of experiments with MAGL and FAAH inhibitors, there already exists evidence that endogenous release of cannabinoid increases in certain some disorders in a way that results in an amelioration of undesirable signs and symptoms (see the section on the endocannabinoid system). As a result, such inhibitors have therapeutic potential.
After anandamide was discovered, the compound most extensively employed to irreversibly suppress its enzymic hydrolysis was phenylmethylsulfonyl fluoride (IC50 for FAAH inhibition = 290 nM to 15 μM), a non-selective serine protease inhibitor that also suppresses MAGL, though less potently (IC50 ≥ 155 μM)100.
Currently, additional inhibitors of FAAH have been discovered5,23,100, where O-1887, URB532, URB597, and AM 374 (the palmitylsulfonyl fluoride equivalent) are most probably the best among these for use as research tools for irreversible FAAH inhibition, as well as OL-135 for reversible FAAH inhibition. All these compounds can bring about their inhibitory effects in vitro at concentrations in the low nanomolar range, which lack the potential to displace radiolabeled ligands from CB1 receptors or (where this has been analyzed) from CB2 receptors. However, it is important to note that the pharmacological characterization of a majority of these inhibitors is incomplete.
The compound O-1887 is a structural equivalent of methyl arachidonyl fluorophosphonate (MAFP), which is an irreversible FAAH inhibitor (IC50 = 1–3 nM) by itself that additionally suppresses both DAGL (IC50 = 800 nM) and MAGL (IC50 = 2–800 nM) but not NAPE-PLD, and potently displaces [3H]CP 55,940 irreversibly from particular binding sites on rat brain membranes (IC50 = 20 nM)5,99,100,104. In one instance, it has been reported that MAFP acts as an irreversible CB1 receptor antagonist118, and in another instance, that it does not54.
Looking now at compounds blocking the metabolism of 2-arachidonylglycerol by suppressing MAGL, several of these have currently been identified99,100,119,120. The most characterized inhibitor of this enzyme is URB602, which induces non-competitive inhibition of MAGL at micromolar concentrations (IC50 = 28 μM) and lacks any noticeable potential to suppress FAAH at 100 μM or to displace [3H]WIN 55,212-2 from CB1 or CB2 receptors at 5 μM119. It has been reported that another compound—URB754—inhibits MAGL with considerably greater potency when compared to URB602120. However, it is now evident that t.his inhibition was produced by an impurity that exists in a commercially available sample of URB754, and the pure compound is deficient of substantial activity as an MAGL inhibitor at concentrations of up to 100 μM (personal communication from Dr Daniele Piomelli).
Inhibitors of the Cellular Uptake of Anandamide
N-(4-hydroxyphenyl) arachidonylamide (AM 404) was the first inhibitor of the cellular uptake of anandamide to be created. Yet, this compound is not specifically selective as it also binds to CB1 receptors, suppresses FAAH, and activates TRPV1 receptors at concentrations at or lesser than those at which it has been found to suppress anandamide uptake. Crucially, it is presently not evident whether any of the compounds identified to suppress the cellular uptake of anandamide thus far do so by targeting an anandamide transport protein or by attenuating FAAH-mediated metabolism of anandamide to induce an intracellular aggregation of this fatty acid amide that is adequate to oppose its penetration into the cell through diffusion25–27.
Some Other Notable Ligands
Apart from the CB1 and CB2 receptor ligands discussed already, there are several other compounds noteworthy because they have the ability to modulate certain effects of standard CB1/CB2 receptor agonists through apparently new mechanisms or because they share the evident potential of such agonists to target specific putative non-CB1 and non-CB2 receptors. These compounds are:
- Δ9-tetrahydrocannabivarin, the plant cannabinoid that displaces [3H]CP 55,940 from CB1 and CB2 receptors at concentrations in the low nanomolar range (Ki = 75.4 and 62.8 nM, respectively) and acts as a CB1 and CB2 receptor competitive antagonist, presenting higher potency against CP 55,940 in the mouse isolated vas deferens and in membranes acquired from human CB2-transfected cells (apparent KB = 10 nM) than in mouse brain membranes (apparent KB = 93 nM)121.
- (–)-Cannabidiol (CBD), the non-psychoactive plant cannabinoid that has therapeutic potential (for example, as an anti-inflammatory agent), lacks substantial affinity for CB1 or CB2 receptors, has anti-oxidant/neuroprotective properties, and blocks, activates, or inhibits (at sub-micromolar concentrations) several standard or putative pharmacological targets including an adenosine transporter122 as well as delayed rectifier K+ and L-type Ca2+ channels, acyltransferase, CYP enzymes, a neuronal non-CB1 site of action in the mouse vas deferens, and the putative non-CB1, non-CB2, and non-TRPV1 “abnormal-CBD receptor” that has been hypothesized to exist in tissues like mesenteric arteries and in microglial cells2,88,123.
- a set of CBD equivalents lacking substantial affinity for the CB1 receptor and acting as antagonists (O-1918) or agonists (abnormal-CBD and O-1602) for the putative abnormal-CBD receptor2,88,96.
- N-arachidonoyl-Lserine, the endogenous compound that may be an endogenous agonist for the abnormal-CBD receptor as it seems to activate this putative receptor upon being added exogenously (EC50 = 550 or ca 1200 nM), and which binds just weakly to CB1 receptors (Ki > 10 μM) and does not bind to TRPV1 or CB2 receptors at concentrations of up to 30 μM124.
- N-vanillyl arachidonyl amide (arvanil), the anandamide/capsaicin structural hybrid that binds to CB1 receptors and suppresses the cellular uptake of anandamide at concentrations in the low micromolar range, binds to TRPV1 receptors at concentrations in the low nanomolar range, and may also have one or more as yet unidentified non-CB1 and non-TRPV1 sites of action125,126.
- Org 27569, Org 29647, and Org 27759, the synthetic indole derivatives, experiments on which have showed the existence of an allosteric site on the cannabinoid CB1 receptor that includes a new target through which CB1 receptor activation could be modulated by endocannabinoids that are endogenously released, for instance, to combat nicotine dependence, obesity, or inflammatory pain127.
It has been observed that although Org 27569, Org 29647, and Org 27759 act as CB1 allosteric enhancers in binding assays, they act as CB1 allosteric inhibitors in functional in vitro bioassays127, thereby restricting their use as experimental tools and establishing a need for additional CB1 allosteric modulators.
There are three other ligands that are worth mentioning: HU 211, the (+)-enantiomer of the potent CB1/CB2 receptor agonist HU 210; Sch.336; and palmitoylethanolamide, the endogenous ligand.
Sch.336, which is a CB2-selective antagonist/inverse agonist, presents much greater potency and efficacy as a CB2 receptor inverse agonist when compared to SR144528128. Sch.336’s high inverse efficacy may be the reason behind its potential to suppress leukocyte migration/trafficking, an effect that could be useful in the clinic for managing inflammatory disorders128.
Although HU 211 lacks substantial affinity for CB1 or CB2 receptors, it has neuroprotective properties that could be due to its potential to reduce the synthesis of tumor necrosis factor-α, to act as a non-competitive antagonist at the N-methyl-D-aspartate (NMDA) receptor, to scavenge oxygen-derived free radicals, and/or to suppress depolarization-evoked calcium fluxes2,129.
Palmitoylethanolamide has gained attention since it lacks substantial affinity for CB1 or CB2 receptors and yet is vulnerable to antagonism by SR144528, a discovery that has led to the postulate that this fatty acid amide could be the endogenous agonist for a “CB2-like” receptor2,88. There is also proof, firstly, that palmitoylethanolamide is a PPAR-α receptor agonist130, secondly that it is metabolized both by PAA and FAAH5 and thirdly, that it may potentiate anandamide via what is called the “entourage effect” (see section on the endocannabinoid system).
Future Directions
The focus of this article has specifically been on ligands that are most extensively used as experimental tools to directly target cannabinoid CB1 and/or CB2 receptors or to modulate endocannabinoids’ tissue levels once they are endogenously released. There is a possibility that future studies in the area of cannabinoid pharmacology will focus on:
- evaluating the therapeutic potential of CB1 and/or CB2 receptor allosteric modulators and neutral antagonists
- investigating the structure-activity relationships of ligands targeting the CB1 allosteric site or acting as neutral CB1 and/or CB2 receptor antagonists
- determining the pharmacological profiles of fresh and current modulators of endocannabinoid biosynthesis, metabolism, or cellular uptake
- collecting more convincing evidence against or for the occurrence of an endocannabinoid transporter in mammalian cells
- characterizing and validating non-CB1 and non-CB2 targets for specific cannabinoids and creating compounds with the ability to selectively activate or block such targets with credible potency
- learn why CB2 receptors appear to be expressed by central neurons
- gaining a more comprehensive knowledge of the role played by the endocannabinoid system in ameliorating the underlying pathology and/or the symptoms of specific disorders
- monitoring early indications for the occurrence of cannabinoid receptors as homodimers or that they form oligomers or heterodimers with one or more classes of non-cannabinoid receptor2
References
- Howlett et al. (2002) Pharmacol. Rev. 54 161.
- Pertwee (2005) In: Cannabinoids. Handbook of Experimental Pharmacology (Vol. 168). Ed. Pertwee, p 1, Springer-Verlag, Heidelberg, Germany.
- Matsuda et al. (1990) Nature 346 561.
- Munro et al. (1993) Nature 365 61.
- Pertwee (2005) AAPS J. 7 E625.
- Valverde et al. (2005). In: Cannabinoids. Handbook of Experimental Pharmacology (Vol. 168). Ed. Pertwee, p 117, Springer-Verlag, Heidelberg, Germany.
- Ashton et al. (2006) Neurosci. Lett. 396 113.
- Gong et al. (2006) Brain Res. 1071 10.
- Van Sickle et al. (2005) Science 310 329.
- Mackie (2005) In: Cannabinoids. Handbook of Experimental Pharmacology (Vol. 168). Ed. Pertwee, p 299, Springer-Verlag, Heidelberg, Germany.
- Pertwee (2001) Progr. Neurobiol. 63 569.
- Walker and Hohmann (2005) In: Cannabinoids. Handbook of Experimental Pharmacology (Vol. 168). Ed. Pertwee, p 509, Springer-Verlag, Heidelberg, Germany.
- Pertwee (1997) Pharmacol. Ther. 74 129.
- Howlett (2005) In: Cannabinoids. Handbook of Experimental Pharmacology (Vol. 168). Ed. Pertwee, p 53, Springer-Verlag, Heidelberg, Germany.
- Calandra et al. (1999) Eur. J. Pharmacol. 374 445.
- Glass and Felder (1997) J. Neurosci. 17 5327.
- Maneuf and Brotchie (1997) Br. J. Pharmacol. 120 1397.
- Breivogel and Childers (2000) J. Pharmacol. Exp. Ther. 295 328.
- Jarrahian et al. (2004) J. Pharmacol. Exp. Ther. 308 880.
- Devane et al. (1992) Science 258 1946.
- Mechoulam et al. (1995) Biochem. Pharmacol. 50 83.
- Sugiura et al. (1995) Biochem. Biophys. Res. Comm. 215 89.
- Di Marzo et al. (2005) In: Cannabinoids. Handbook of Experimental Pharmacology (Vol. 168). Ed. Pertwee, p 147, Springer-Verlag, Heidelberg, Germany.
- Cravatt and Lichtman (2004) J. Neurobiol. 61 149.
- Hillard and Jarrahian (2003) Br. J. Pharmacol. 140 802.
- Kaczocha et al. (2006) J. Biol. Chem. 281 9066.
- Alexander and Cravatt (2006) J. Am. Chem. Soc. 128 9699.
- Cravatt et al. (1996) Nature 384 83.
- Cravatt et al. (2001) Proc. Natl. Acad. Sci. USA 98 9371.
- Cravatt et al. (2004) Proc. Natl. Acad. Sci. USA 101 10821.
- Okamoto et al. (2004) J. Biol. Chem. 279 5298.
- Karlsson et al. (2001) Gene 272 11.
- Dinh et al. (2002) Proc. Natl. Acad. Sci. USA 99 10819.
- Ho et al. (2002) J. Biol. Chem. 277 1816.
- Bisogno et al. (2003) J. Cell Biol. 163 463.
- Leung et al. (2006) Biochemistry 45 4720.
- Mechoulam et al. (1998) Eur. J. Pharmacol. 359 1.
- Vaughan and Christie (2005) In: Cannabinoids. Handbook of Experimental Pharmacology (Vol. 168). Ed. Pertwee, p 367, Springer-Verlag, Heidelberg, Germany.
- Walter and Stella (2004) Br. J. Pharmacol. 141 775.
- Cabral and Staab (2005) In: Cannabinoids. Handbook of Experimental Pharmacology (Vol. 168). Ed. Pertwee, p 385, Springer-Verlag, Heidelberg, Germany.
- Pertwee (1999) Curr. Med. Chem. 6 635.
- Bayewitch et al. (1996) J. Biol. Chem. 271 9902.
- Steffens et al. (2005) Biochem. Pharmacol. 69 169.
- Savinainen et al. (2005) Br. J. Pharmacol. 145 636.
- Abadji et al. (1994) J. Med. Chem. 37 1889.
- Pertwee (1988) Pharmacol. Ther. 36 189.
- TocrisolveTM 100 (2006) In: Life Science Catalogue p 459, Tocris Bioscience, Bristol, UK.
- Pertwee et al. (2000) Br. J. Pharmacol. 129 1577.
- Di Marzo et al. (2001) Biochem. Biophys. Res. Comm. 281 444.
- Hillard et al. (1999) J. Pharmacol. Exp. Ther. 289 1427.
- Jarrahian et al. (2000) J. Neurochem. 74 2597.
- Hanus et al. (2001) Proc. Natl. Acad. Sci. USA 98 3662.
- Savinainen et al. (2001) Br. J. Pharmacol. 134 664.
- Savinainen et al. (2003) Br. J. Pharmacol. 140 1451.
- Ruiu et al. (2003) J. Pharmacol. Exp. Ther. 306 363.
- Rinaldi-Carmona et al. (1994) FEBS Lett. 350 240.
- Felder et al. (1998) J. Pharmacol. Exp. Ther. 284 291.
- Felder et al. (1995) Mol. Pharmacol. 48 443.
- Showalter et al. (1996) J. Pharmacol. Exp. Ther. 278 989.
- Lan et al. (1999) AAPS PharmSci 1 U13.
- Lan et al. (1999) J. Med. Chem. 42 769.
- Lin et al. (1998) J. Med. Chem. 41 5353.
- Khanolkar et al. (1996) J. Med. Chem. 39 4515.
- Goutopoulos et al. (2001) Bioorg. Med. Chem. 9 1673.
- Ibrahim et al. (2003) Proc. Natl. Acad. Sci. USA 100 10529.
- Huffman et al. (1999) Bioorg. Med. Chem. 7 2905.
- Valenzano et al. (2005) Neuropharmacology 48 658.
- Hanus et al. (1999) Proc. Natl. Acad. Sci. USA 96 14228.
- Rinaldi-Carmona et al. (1998) J. Pharmacol. Exp. Ther. 284 644.
- Iwamura et al. (2001) J. Pharmacol. Exp. Ther. 296 420.
- Ross et al. (1999) Br. J. Pharmacol. 126 665.
- Pertwee (2000) Exp. Opin. Invest. Drugs 9 1553.
- Brusa et al. (2006) Int. Cann. Res. Soc. Abstr. 42.
- Pertwee (2005) Life Sci. 76 1307.
- Ross et al. (1999) Br. J. Pharmacol. 128 735.
- Hosohata et al. (1997) Life Sci. 61 PL115.
- Hosohata et al. (1997) Eur. J. Pharmacol. 321 R1.
- Pertwee et al. (1996) Br. J. Pharmacol. 118 2199.
- Landsman et al. (1998) Life Sci. 62 PL109.
- Vásquez et al. (2003) Can. J. Physiol. Pharmacol. 81 436.
- Gatley et al. (1997) Life Sci. 61 PL191.
- Gatley et al. (1998) J. Neurochem. 70 417.
- Lan et al. (1996) J. Labelled Comp. Radiopharm. 38 875.
- Barth (1998) Exp. Opin. Ther. Patents 8 301.
- Lindsey et al. (2005) In: Cannabinoids. Handbook of Experimental Pharmacology (Vol. 168). Ed. Pertwee, p 425, Springer-Verlag, Heidelberg, Germany.
- Oz (2006) Pharmacol. Ther. 111 114.
- Cheer et al. (1999) Neuropharmacology 38 533.
- Pertwee (2004) Curr. Neuropharmacol. 2 9.
- Hájos and Freund (2002) Neuropharmacology 43 503.
- Breivogel et al. (2001) Mol. Pharmacol. 60 155.
- Barann et al. (2002) Br. J. Pharmacol. 137 589.
- Bradshaw and Walker (2005) Br. J. Pharmacol. 144 459.
- Pertwee (2006) Int. J. Obes. 30 S13.
- O’Sullivan et al. (2005) Biochem. Biophys. Res. Comm. 337 824.
- O’Sullivan et al. (2006) J. Pharmacol. Exp. Ther. 317 428.
- Pacher et al. (2005) In: Cannabinoids. Handbook of Experimental Pharmacology (Vol. 168). Ed. Pertwee, p 599, Springer-Verlag, Heidelberg, Germany.
- Sjögren et al. (2005) Int. Cann. Res. Soc. Abstr. 106.
- Brown et al. (2005) Int. Cann. Res. Soc. Abstr. 16.
- Bisogno et al. (2006) Biochim. Biophys. Acta 1761 205.
- Ho and Hillard (2005) In: Cannabinoids. Handbook of Experimental Pharmacology (Vol. 168). Ed. Pertwee, p 187, Springer-Verlag, Heidelberg, Germany.
- Deutsch et al. (1997) Biochem. Biophys. Res. Comm. 231 217.
- Lang et al. (1996) Anal. Biochem. 238 40.
- De Petrocellis et al. (1997) Biochem. Biophys. Res. Comm. 231 82.
- Deutsch et al. (1997) Biochem. Pharmacol. 53 255.
- Martin et al. (2000) J. Pharmacol. Exp. Ther. 294 1209.
- Kathuria et al. (2003) Nature Med. 9 76.
- Lichtman et al. (2004) J. Pharmacol. Exp. Ther. 311 441.
- Jonsson et al. (2001) Br. J. Pharmacol. 133 1263.
- Koutek et al. (1994) J. Biol. Chem. 269 22937.
- Sheskin et al. (1997) J. Med. Chem. 40 659.
- Huang et al. (2001) J. Biol. Chem. 276 42639.
- Ortar et al. (2003) Biochem. Pharmacol. 65 1473.
- Fowler et al. (2004) Eur. J. Pharmacol. 492 1.
- De Petrocellis et al. (2000) FEBS Lett. 483 52.
- López-Rodríguez et al. (2003) Eur. J. Med. Chem. 38 403.
- Beltramo et al. (1997) Science 277 1094.
- Bisogno et al. (2001) Br. J. Pharmacol. 134 845.
- Fernando and Pertwee (1997) Br. J. Pharmacol. 121 1716.
- Hohmann et al. (2005) Nature 435 1108.
- Makara et al. (2005) Nature Neurosci. 8 1139.
- Thomas et al. (2005) Br. J. Pharmacol. 146 917.
- Carrier et al. (2006) Proc. Natl. Acad. Sci. USA 103 7895.
- Pertwee (2004). In: Cannabinoids. Ed. Di Marzo, p 32. Kluwer Academic/Plenum Publishers, New York, USA.
- Milman et al. (2006) Proc. Natl. Acad. Sci. USA 103 2428.
- Melck et al. (1999) Biochem. Biophys. Res. Comm. 262 275.
- Di Marzo et al. (2000) Eur. J. Pharmacol. 406 363.
- Price et al. (2005) Mol. Pharmacol. 68 1484.
- Lunn et al. (2006) J. Pharmacol. Exp. Ther. 316 780.
- Pertwee (2000) Addict. Biol. 5 37.
- Lo Verme et al. (2005) Mol. Pharmacol. 67 15.
- Moore et al. (2005) Proc. Natl. Acad. Sci. USA 102 17852.
About Tocris Bioscience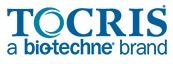
Tocris Bioscience is your trusted supplier of high-performance life science reagents, including receptor agonists & antagonists, enzyme inhibitors, ion channel modulators, fluorescent probes & dyes, and compound libraries. Our catalog consists of over 4,500 research tools, covering over 400 protein targets enabling you to investigate and modulate the activity of numerous signaling pathways and physiological processes.
We have been working with scientists for over 30 years to provide the life science community with research standards, as well as novel and innovative research tools. We understand the need for researchers to trust their research reagents, which is why we are committed to supplying our customers with the highest quality products available, so you can publish with confidence.
Tocris is part of the protein sciences division of Bio-Techne, which also includes the best in class brands R&D Systems, Novus Biologicals, ProteinSimple, and Advanced Cell Diagnostics. Bio-Techne has united these brands to provide researchers with a full portfolio of research reagents, assays, and protein platforms. For more information on Bio-Techne and its brands, please visit bio-techne.com.
Sponsored Content Policy: News-Medical.net publishes articles and related content that may be derived from sources where we have existing commercial relationships, provided such content adds value to the core editorial ethos of News-Medical.Net which is to educate and inform site visitors interested in medical research, science, medical devices, and treatments.