In the late 1950s, dopamine was recognized as a neurotransmitter in its own right. A specific functional role for dopamine was suggested by the demonstration of its uncharacteristic distribution in the brain, separate from the distribution of noradrenaline.1 The realization that dopamine played a significant role in the pathogenesis or drug treatment of certain disorders, such as Parkinson’s disease and schizophrenia, intensified the interest in dopamine.2,3
Much research followed on the sites of action of dopamine and the dopamine receptors. Cools and van Rossum suggested one milestone based upon anatomical, electrophysiological and pharmacological studies: namely that there might indeed exist more than one type of receptor for dopamine within the brain.4
Biochemical studies of the 1970s on dopamine receptors, which were based on second messenger assays (e.g. stimulation of cAMP production and ligand binding assays) corroborated this concept, which was subsequently gifted a firm foundation by Kebabian and Calne’s 1979 review.5 Elaborating on an earlier suggestion by Spano et al.6, the pair proposed the existence of two classes of dopamine receptor, D1 and D2, which each had different biochemical and pharmacological properties, and which mediated different physiological functions. To define the two subtypes in functional assays, selective agonists and antagonists exist. Both the D1 and D2 subtypes are G protein-coupled receptors (GPCRs); however, divergent G proteins and effectors are involved in each of their signaling pathways (Figure 1).
Despite some indications of further heterogeneity of these dopamine receptor subtypes in biochemical studies, the true extent of this remained unknown until the late 1980s, when it was revealed with the application of gene cloning techniques. The studies showed that there are a minimum of five dopamine receptors (D1– D5) that may be then divided into two subfamilies, the properties of which resemble either the original D1 and D2 receptors.7,8 The receptor family termed ‘D1-like’, comprises D1 and D5 and corresponds to the original D1 receptors, whereas the ‘D2-like’ receptor family is comprised of D2, D3 and D4 receptors and therefore corresponds to the original D2 receptors.
Throughout this article, receptor subtypes defined from cloned genes are referred to as D1, D2, D3, D4, D5, and where only the subfamily of receptor has been defined pharmacologically the terms used are the D1-like and D2-like nomenclature.
Properties of the Dopamine Receptor Subtypes
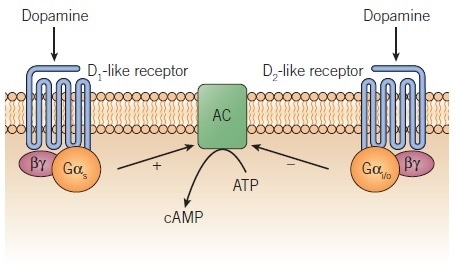
Figure 1. Regulation of adenylyl cyclase by D1-like and D2-like dopamine receptors. Image credit: Tocris Bioscience
Common Receptor Properties
Significant homologies have been demonstrated to exist among the subtypes thanks to analysis of the amino acid sequences of the dopamine receptor subtypes, with the greatest found between members of either subfamily.7,8 Seven stretches of amino acids have been shown contained within each receptor, which are hydrophobic and long enough to span the membrane. It therefore appears that each of the dopamine receptors adheres to the general structural model for a GPCR.9–11 Each, therefore, possesses an extracellular amino terminus and seven putative membranes spanning α-helices connected by intracellular and extracellular protein loops (Figure 2).
The amino terminus and second extracellular loop offer one or more potential sites for glycosylation. The helices are grouped together in the membrane, which forms the ligand-binding site (Figure 2); some information exists regarding the residues that make contact with ligands.11,12 There is also an intracellular carboxyl terminus, which likely bears a palmitoyl group and may form an additional link to the membrane.
The D1-like receptors have long carboxyl terminal tails and short third intracellular loops, while the D2-like receptors have short carboxyl terminal tails and long third intracellular loops. In addition to offering a structural basis for the bifurcation of the receptors into their subfamilies, this is also likely to have a functional significance, potentially related to the specificity of receptor/G protein interaction. The third intracellular loop, which is herein termed ‘I3’, is significant for the relationship between the receptor and G protein. Regarding the D2-like receptors, variants of the subtypes exist based upon this intracellular loop. For instance, there are both long and short splice variants of the D2 and D3 receptors with the long forms having an insertion (29 amino acids for the long D2, D2L) in this loop.13,14 Polymorphic variants of the D2 receptor have been recorded, which display single amino acid changes in I3.15 In the human population, the D4 receptor is highly polymorphic with variants containing different length insertions in I3.16,17 In certain instances, these D2-like receptor variants may possess divergent abilities to couple to or activate G proteins,18,19 and can also display differing pharmacological properties.16,20,21
In certain developed lines of mice, the I3 insertion that produces the long D2 receptor variant (D2L) is deleted, which results in the expression of the short variant (D2S) only. Subsequent analysis of the mice indicates that the splice variants are not fully interchangeable; in mice that express only D2s 22-24, some D2 receptor responses are not observed in mice. The D4 receptor variants have not been found to demonstrate any substantive differences in either agonist signaling or in coupling to G proteins.25
The unique properties of these varying subtypes were initially explored by expressing the receptors in recombinant cells and analyzing the localization of the subtypes at the mRNA and protein level. Latterly, further study of these receptors has been enabled by the use of subtype-selective drugs and transgenic mice, with one or more receptor subtypes genetically deleted.
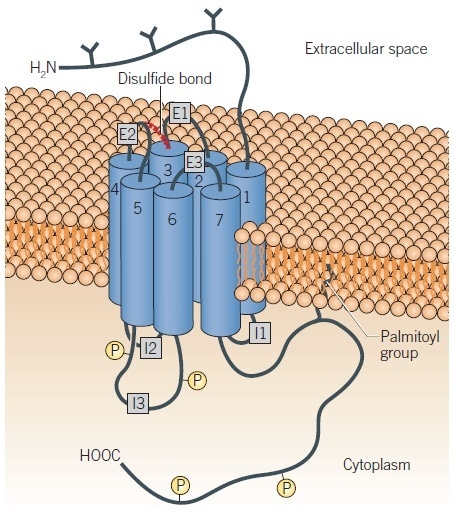
Figure 2. Schematic representation of a G protein-coupled dopamine receptor. Image credit: Tocris Bioscience
Individual Receptor Properties
Different properties are exhibited by the dopamine receptor subtypes, in terms of their pharmacological profile, localization, and mechanism of action. The section below will briefly summarize these key differences.
D1-like Receptors
The D1 and D5 receptors both display similar pharmacological properties to those displayed by the original pharmacologically defined D1 receptor. These include a high affinity for the benzazepine ligands SCH 23390, SCH 39166, and SKF 83566, all selective antagonists for these subtypes. LE 300 is a potent D1-like antagonist that is useful thanks to its structural distinctiveness from the benzazepines, though it is not as selective for D1 over D2 as the benzazepine antagonists. Thioxanthines such as flupentixol and phenothiazines like fluphenazine also demonstrate this strong affinity; however, they are not selective for D1-like over D2-like receptors.
The development of SKF 38393, the first D1-like receptor agonist, held significance for the differentiation between activation of D1-like and D2-like receptors, although it was later understood that the partial agonist nature of SKF 38393 produced an under-appreciation of the contribution of D1-like receptors to behavior.26 The D1-like receptors display moderate affinities for typical dopamine agonists such as apomorphine; full and/or selective D1-like receptor agonists such as A 77636, A 68930, SKF 81297, dihydrexidine, and doxanthrine are now available. Some minor differences exist in the affinities of some compounds for the D1 and D5 receptors (higher agonist and lower antagonist affinities for D5), but no compounds that decisively distinguish between those subtypes are currently available.27,28
The typical dopamine-rich areas of the brain such as the neostriatum, substantia nigra, nucleus accumbens, and olfactory tubercle are most commonly where D1 receptors are found. This is in contrast to the distribution of the D5 receptors, which is much more restricted; generally, this subtype is located at much lower levels. Both receptors can stimulate adenylyl cyclase (Figure 1), with the D5 receptor showing some constitutive activity for this response.28 Recombinant systems show inverse agonist activity at the D1 and D5 receptors for some compounds such as butaclamol,28 compounds similar to which were previously considered to be antagonists.
For some time, it has been known that stimulation of a D1-like receptor leads to activation of phospholipase C29 – a response which has recently been connected to the D1/D2 receptor heterodimer, offering a function for heterodimer formation.30 Those agonists that preferentially stimulate the cAMP response (like SKF 83822) or the phospholipase C response (like SKF 83959) associated with D1-like receptors have been recorded.31
It seems that the D1 receptor mediates significant actions of dopamine, to control movement, cognitive function, and cardiovascular function. Direct interactions between ion channel-linked receptors and D1-like receptors have occurred (D1 / NMDA, D5 /GABAA ),32 which leads to modulation of receptor function. Such mechanisms provide for cross-talk between slow and fast neurotransmitter systems, and may in addition indicate a further functional role for the little-understood D5 receptor. It has been suggested, by studies with null mutant ‘knock-out’ mice, that in certain ways the D1 and D5 receptor functions are reciprocal. For instance, as regards to spontaneous locomotion – and in other respects similar, such as with respect to grooming or psychostimulant-induced locomotion.33,34
D2-like Receptors
Overall, the pharmacological properties exhibited by the D2, D3 and D4 are similar to those exhibited by the originally defined D2 receptor. That is to say, all the above display high affinities for drugs such as the butyrophenones (haloperidol, spiperone) and substituted benzamides (sulpiride, raclopride), and these classes of drugs provide selective antagonists for D2-like receptors over D1-like receptors.
As demonstrated above, the D2-like receptors display high affinities for phenothiazines and thioxanthines. Every D2-like receptor possesses its own pharmacological signature, which means that certain differences exist in affinities of drugs for the individual D2-like receptors. For instance, the antagonists sulpiride and raclopride show high affinity for the D2 and D3 receptors but have a lower affinity for the D4 receptor. Clozapine displays a moderate selectivity as regards to the D4 receptor.
The majority of D2 antagonists have a higher affinity for the D2 receptor in comparison with the D3 and D4 receptors. This occurs as the D2 receptor, which has a much greater presence within the brain than the other D2-like receptors, corresponds to the receptor for which such drugs were developed. The development of more selective antagonists that are invaluable in determining the functions of these subtypes was enabled by the molecular cloning of additional D2-like receptors. For instance, L-741,626, NGB 2904, and L-745,870 are D2 selective (~40- fold), D3 selective (~200-fold) and D4 selective (~2000-fold) antagonists, respectively.35,36
Certain selective agonists for the D2-like receptors relative to the D1-like receptors, e.g. N-0437, PHNO, and quinpirole, have been created. Sumanirole is a full-efficacy agonist that, over other dopamine receptors, is highly selective for the D2 receptor.37 A number of D4 agonists of varying efficacy and selectivity exist, with A 412997 being an example of a highly selective full D4 agonist.38 Multiple agonists seem to be highly selective for the D3 receptor over other D2-like receptors in radioligand binding assays. However, this frequently offers an invalid comparison between a mixed population of agonist high- and low‑affinity D2 receptors, and the D3 receptor that is apparently restricted in an agonist-high affinity conformation,39 rather than a difference in drug concentrations, which can activate the two subtypes.40 When functional responses or binding are compared to the agonist high-affinity state of the receptors, it is determined that dopamine and some agonists such as pramipexole are modestly D3 selective.41
The prominent D2-like subtype in the brain is the D2 receptor, which is found at high levels in typical areas of the brain that are dopamine-rich. The receptors D3 and D4 are located at much lower levels and in a more constrained pattern of distribution, largely in limbic areas of the brain. Certain D3 receptors can also be pinpointed in motor-function-related regions like the putamen.
The D2-like receptor subtypes have each been proven to inhibit adenylyl cyclase (Figure 1) when expressed in recombinant cells,40,42,43, although the signal via the D3 receptor is harder to demonstrate and is generally lower than it is for the other two subtypes. Preferential coupling of the D3 receptor to specific adenylyl cyclase isoforms may explain this.44 Upon activation, the D2-like receptors will stimulate a variety of processes including acute signaling events (inhibition of adenylyl cyclase, stimulation of K+ channels, inhibition of Ca2+ channels, stimulation of arachidonic acid release) as well as longer-term events (MAP kinase and β-arrestin-2/Akt/GSK-3 signaling, and mitogenesis).45,46
D3 receptor-mediated signaling events often have a higher magnitude than for the other D2-like receptors. We are just beginning to clarify the relation of these signaling events to in vivo responses. Multiple compounds that were thought to be antagonists at D2-like receptors (including the antipsychotic drugs haloperidol, chlorpromazine, and clozapine) have been demonstrated to possess inverse agonist activity at D2 and D3 receptors.47–49 This inverse agonism may contribute to the increases in D2 receptor number displayed by the brain when these drugs are used to treat experimental animals chronically.
The D2 receptor’s functions are significant when it comes to certain aspects of brain behavior, mediating the effects of dopamine to control movement, and prolactin secretion from the anterior pituitary gland. D3 and D4 receptor functions are not yet known, though roles in cognitive, emotional and behavioral function are suggested by their localizations in limbic areas of the brain. Thanks to these properties, they are attractive targets when it comes to designing potential selective antipsychotic drugs. Most drugs used to treat schizophrenia (antipsychotics) and Parkinson’s (e.g. bromocriptine) share high affinities with the D2‑like receptors.3 L-745,870 was the first highly selective D4 antagonist synthesized, which eventually proved to be inactive against psychosis inherent in schizophrenia.50
Future Directions
Understanding the Role of Dopamine Receptor Subtypes
Understanding the role of these different receptor subtypes is still a long way off. Though partially selective antagonists and transgenic ‘receptor knock out’ animals are available for some of these subtypes, there is much still to be done (see for example Waddington et al (2005)31).
Dopamine Receptor Subtypes in Drug Discovery
Dopamine receptors enjoy a status as a popular target for drug discovery campaigns, thanks to the importance of dopamine for the pathogenesis or drug treatment of several significant disorders, such as Parkinson’s disease, schizophrenia and pituitary prolactin dysfunction. Research continues in these areas and has since expanded into departments like drug dependence and penile erectile dysfunction.
A new avenue of exploration that has been explored in the lane of effective antipsychotics is the development of dopamine D2-like partial agonists, like aripiprazole.51 Previously, it had been thought that antipsychotics had to be antagonists / inverse agonists at the D2 receptor.3 However, the effective use of D2 partial agonists as antipsychotics invites questions regarding the mechanics of D2 receptor-mediated antipsychotic activity that still need to be addressed.52 For instance, it will be significant to understand the relationship between the efficacy of the ligands in signaling assays and their therapeutic effects.
The availability of crystal structures for the D3 receptor and the β2-adrenoceptor is a significant new tool for the rational design of subtype-selective drugs.10,11 These structures (as well as the precise models which can be generated on the basis of their close homology with other D2-like receptors and the D1-like receptor, respectively) permit the prediction of the affinity of a receptor for molecules that may be novel scaffolds.53,54
Biochemical Mechanisms Underling the Effects of Dopamine Receptor Activation
It will soon be vital to clearly define how changes in the functions of cells like neurons are caused by activation of dopamine receptors. This will, in time, furnish us with a better understanding of how drugs work in and impact the brain, investigation into which area has already begun. For instance, it has been demonstrated in striatal neurons that a protein strongly regulated by dopamine receptors is DARPP-32 (dopamine and cAMP-regulated phosphoprotein 32 kD), which appears to be a key protein in striatal neuronal function.55
Significant progress in understanding temporal aspects of brain signalling processes has also been made. There may be, it has been suggested, two waves of dopamine-mediated responses: one set of faster responses associated with changes in cAMP and DARPP-32 phosphorylation, and another slower set of non-cAMP mediated processes associated with β-arrestin-2/Akt/GSK-3 signaling.46
Interactions of Dopamine Receptors With Other Proteins
The interactions of dopamine receptors with other proteins are, it is clear, a key step when it comes to determining their function. This interaction represents an imminently active research field of the near future. The group including cytoskeletal, adapter, and signaling proteins that interact with dopamine receptors have been named DRIPs, which stands for dopamine receptor-interacting proteins.56 An example of this is the neuronal Ca2+ sensor-I (NCS-1), which interacts with D2.57 Herein, the interacting proteins may impact the effects on the D2 Receptor of Ca2+. Interactions with other receptors also occur, including ligand-gated ion channels, as well as GPCRs leading to homo- and heterodimer formation. A role has been suggested for D1/D2 heterodimer formation,58 and roles for homodimer formation, e.g. D2 homodimers,59,60 are currently being actively developed.
Development and Characterization Of Biased Ligands
An observation that holds potential for drug development is the fact that many ligands do not engage (to the same extent) all mechanisms which are consequences of activation of a receptor. Activation of a receptor by its endogenous agonist may activate G protein- and β-arrestin-mediated signaling, phosphorylation of the receptor by one or more protein kinases, desensitization, and receptor internalization, which is then followed by either recycling or degradation of the receptor. For some of these responses, a given ligand may be fully efficacious (i.e., a full agonist) and a partial agonist (or an antagonist) at other responses mediated by the same receptor.61,62 This is referred to as ‘functional selectivity’ as a phenomenon, and a ligand that engages only some of the responses available to a receptor is a functionally selective (or, in other words, ‘biased’) ligand.
Parkinson’s disease has a promising target for treatment in the D1 receptor. Two obstacles against the use of D1 agonists are the rapid development of tolerance as well as the side effects of hypotension and seizures.63 Both impediments could be addressed through developing biased agonists. For instance, SKF 83822 is a functionally selective ligand biased towards D1-mediated signaling via adenylyl cyclase, that additionally causes seizures at high doses,64 while SKF 83959 preferentially activates the D1 /D2 receptor heteromer and the associated phospholipase C response.30
Biased ligands (which are biased towards either G protein- or β-arrestin-mediated signaling pathways modulated by the D2 receptor) are also developmentally interesting, as they hold potential benefits as pharmacological tools and potential antipsychotic drugs that may have fewer side effects or be more efficacious.
References
- Carlsson et al. (1958) Science 15 471.
- Hornykiewicz (1973) Fed.Proc. 32 183.
- Strange (2001) Pharmacol.Rev. 53 119.
- Cools and van Rossum (1976) Psychopharmacologia 45 243.
- Kebabian and Calne (1979) Nature 277 93.
- Spano et al. (1978) Adv.Biochem.Psychopharm. 19 155.
- Missale et al. (1998) Physiol.Rev. 78 189.
- Neve and Neve (1997) Molecular biology of dopamine receptors. in: Neve and Neve eds. The Dopamine Receptors. Humana Press, Totawa, NJ.27.
- Palczewski et al. (2000) Science 289 739.
- Kobilka and Schertler (2008) Trends Pharmacol.Sci. 29 79.
- Chien et al. (2010) Science 330 1091.
- Ballesteros et al. (2001) Mol.Pharmacol. 60 1.
- Giros et al. (1989) Nature 342 923.
- Fishburn et al. (1993) J.Biol.Chem. 268 5872.
- Cravchik et al. (1996) J.Biol.Chem. 271 26013.
- Van Tol et al. (1992) Nature 358 149.
- Seeman and Van Tol (1994)Trends Pharmacol.Sci. 15 264.
- Castro and Strange (1993) FEBS Lett. 315 223.
- Guiramand et al. (1995) J.Biol.Chem. 270 7354.
- Castro and Strange (1993) J.Neurochem. 60 372.
- Malmberg et al. (1993) Mol.Pharmacol. 43 749.
- Wang et al. (2000) J.Neurosci. 20 8305.
- Usiello et al. (2000) Nature 408 199.
- Lindgren et al. (2003) Proc.Natl.Acad.Sci.U.S.A. 100 4305.
- Kazmi et al. (2000) Biochemistry 39 3734.
- Setler et al. (1978) Eur.J.Pharmacol. 50 419.
- Sunahara et al. (1991) Nature 350 614.
- Tiberi and Caron (1994) J.Biol.Chem. 269 27925.
- Undie et al. (1994) J.Neurochem. 62 2045.
- Rashid et al. (2007) Proc.Natl.Acad.Sci.U.S.A. 104 654.
- Waddington et al. (2005) Psychopharmacology (Berl). 181 611.
- Salter (2003) Trends Neurosci. 26 235.
- O’Sullivan et al. (2010) Dopamine receptors and behavior: from psychopharmacology to mutant models. in: Neve ed. The Dopamine Receptors, 2nd Edition. Humana Press, New York, NY. 323.
- Holmes et al. (2001) Behav.Neurosci. 115 1129.
- Kulagowski et al. (1996) J.Med.Chem. 39 1941.
- Yuan et al. (1998) Bioorg.Med.Chem.Lett. 8 2715.
- Mccall et al. (2005) J.Pharmacol.Exp.Ther. 314 1248.
- Moreland et al. (2005) Pharmacol.Biochem.Behav. 82 140.
- Vanhauwe et al. (2000) J.Pharmacol.Exp.Ther. 295 274.
- Chio et al. (1994) Mol.Pharmacol. 45 51.
- Mierau et al. (1995) Eur.J.Pharmacol. 290 29.
- Gardner et al. (1996) Br.J.Pharmacol. 118 1544.
- Tang et al. (1994) J.Pharmacol.Exp.Ther. 268 495.
- Robinson and Caron (1997) Mol.Pharmacol. 52 508.
- Neve et al. (2004) J.Recept.Signal.Transduct.Res. 24 165.
- Beaulieu et al. (2007) Trends Pharmacol.Sci. 28 166.
- Hall and Strange (1997) Br.J.Pharmacol. 121 731.
- Griffon et al. (1996) J.Neural Transm. 103 1163.
- Akam and Strange (2004) Biochem.Pharmacol. 67 2039.
- Bristow et al. (1997) Trends Pharmacol.Sci. 18 186.
- Swainston and Perry (2004) Drugs. 64 1715.
- Strange (2008) Trends Pharmacol.Sci. 29 314.
- Kufareva et al. (2011) Structure. 19 1108.
- Carlsson et al. (2011) Nat.Chem.Biol. 7 769.
- Svenningsson et al. (2004) Annu.Rev.Pharmacol.Toxicol. 44 269.
- Hazelwood et al. (2010) Dopamine receptor-interacting proteins. in: Neveed.The Dopamine Receptors. Humana Press, New York, NY. 219.
- Woll et al. (2011) Biochemistry 50 8780.
- Hasbi et al. (2009) Proc.Natl.Acad.Sci.U.S.A. 106 21377.
- Armstrong and Strange (2001) J.Biol.Chem. 276 22621.
- Lee et al. (2000) Mol.Pharmacol. 58 120.
- Urban et al. (2007) J.Pharmacol. Exp.Ther. 320 1.
- Neve (2009) Functional Selectivity of G Protein-Coupled Receptors: New Opportunities for Drug Discovery. Humana Press, NewYork,NY.
- Mailman et al. (2001) Curr.Opin.Investig.Drugs. 2 1582.
- O’Sullivan et al. (2004) Eur.J.Pharmacol. 486 273.
- Monsma et al. (1990) Proc.Natl.Acad.Sci.U.S.A 87 6723.
- Bunzow et al. (1988) Nature 336 783.
- Sokoloff et al. (1990) Nature 347 146.
- Van Tol et al. (1991) Nature 350 610.
- Jarvie and Caron (1993) Adv.Neurol. 60 325.
- Wu et al. (2005) J.Med.Chem. 48 680.
About Tocris Bioscience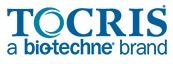
Tocris Bioscience is your trusted supplier of high-performance life science reagents, including receptor agonists & antagonists, enzyme inhibitors, ion channel modulators, fluorescent probes & dyes, and compound libraries. Our catalog consists of over 4,500 research tools, covering over 400 protein targets enabling you to investigate and modulate the activity of numerous signaling pathways and physiological processes.
We have been working with scientists for over 30 years to provide the life science community with research standards, as well as novel and innovative research tools. We understand the need for researchers to trust their research reagents, which is why we are committed to supplying our customers with the highest quality products available, so you can publish with confidence.
Tocris is part of the protein sciences division of Bio-Techne, which also includes the best in class brands R&D Systems, Novus Biologicals, ProteinSimple, and Advanced Cell Diagnostics. Bio-Techne has united these brands to provide researchers with a full portfolio of research reagents, assays, and protein platforms. For more information on Bio-Techne and its brands, please visit bio-techne.com.
Sponsored Content Policy: News-Medical.net publishes articles and related content that may be derived from sources where we have existing commercial relationships, provided such content adds value to the core editorial ethos of News-Medical.Net which is to educate and inform site visitors interested in medical research, science, medical devices, and treatments.