Neurotransmission, or synaptic transmission, corresponds to the movement of signals between neurons, permitting the transmission of information through the proliferation of action potentials.
This procedure is the foundation of inter-neuron communication within, and between, the peripheral and central nervous systems, and is essential for memory and cognition, muscle contraction, and co-ordination of organ function.
The summary below delineates the principles of dopaminergic, opioid, glutamatergic, and serotonergic transmission, while also supplying a short description of how neurotransmission can be examined among an array of neurological disorders.
In addition, the guide also presents essential products for the investigation of neurotransmission, targeting divergent neurotransmitter systems. The utilization of small molecules to study neuronal circuits has engendered an enhanced awareness of the fundamental mechanisms of disease states related to neurotransmission, while also highlighting new directions for treatment.
Tocris offers a cutting-edge selection of high-performance life science reagents for use in neurotransmission research, furnishing operators with the most innovative tools to interrogate neuronal network signaling in health and disease.
Principles of Neurotransmission
The preponderance of neurotransmission transpires across chemical synapses, among which an endogenous neurotransmitter is released by the presynaptic neuron and is subsequently identified by receptors on the postsynaptic neuron (Figure 1).
Broadly speaking, neurotransmitters can be differentiated into three classes; amino acids comprising glutamate and glycine, amines comprising dopamine (DA), serotonin (5-HT) and norepinephrine (NE), as well as peptides such as dynorphin, the enkephalins and neuropeptide Y. Whereas the amino acids glycine and glutamate are located in all bodily cells, other neurotransmitters are only synthesized by neurons.
After synthesis, neurotransmitters are taken up and collected in synaptic vesicles, in preparation for release. The release of a neurotransmitter is activated by the introduction of action potentials in the axon terminal of the presynaptic neuron, causing voltage-gated Ca2+ channels to open, and permitting an influx of ions.
The consequent increase in intracellular Ca2+ concentration provokes synaptic vesicles to combine with the presynaptic membrane, bringing about the release of the neurotransmitter into the synaptic cleft through exocytosis.
Neurotransmitters traverse the synaptic cleft and cohere to their particular receptors. These might be ligand-gated ion channels (LGICs) or G protein-coupled receptors (GPCRs), while certain neurotransmitters possess receptors in each category.
The cohering of a neurotransmitter to an LGIC engenders a conformational alteration in the protein's structure, permitting the movement of ions through the channel. The movement of ions through channels that are selective for positively charged cations causes the postsynaptic membrane to depolarize and an action potential to initiate in the postsynaptic neuron.
Contrastingly, the movement of ions through negatively charged, anion-selective channels causes hyperpolarization of the postsynaptic membrane, thus restricting action potential inception. The cohering of a neurotransmitter to a GPCR brings about the activation of G proteins, which are subsequently able to trigger enzymes into modulating intracellular signaling pathways. The ultimate consequence of this is the modulation of the activity of other proteins such as ion channels and enzymes.
As soon as a neurotransmitter has cohered to its receptor, it is removed from the synaptic cleft to permit an additional occurrence of synaptic transmission. Neurotransmitter molecules are taken up by the presynaptic neuron, or by alternative cell types such as astrocytes, via specific reuptake transporters.
Neurotransmitters can subsequently undergo metabolization, to be reused for further generation, or can be recycled into synaptic vesicles.
The robustness of the synaptic connection between two neurons is dependent on an assortment of variables. These comprise the size of the post-synaptic potential generated by binding of the neurotransmitter to its receptor, the number of individual synapses between two neurons and the probability of neurotransmitter release at the presynaptic membrane.
The existence of neurotransmitter receptors on the pre-synaptic membrane also controls the release of neurotransmitters, via both positive and negative feedback loops. Synaptic connection robustness is an essential component in cognitive operations such as memory formation.
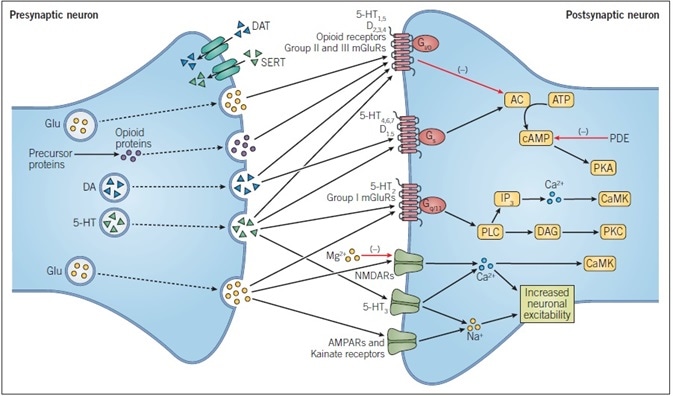
Figure 1. Principles of Neurotransmission. This simplified schematic shows the main events during dopaminergic, glutamatergic, opioid peptide, and serotonergic neurotransmission. DA and 5-HT are both biogenic amines that are derived from amino acids, while glutamate itself is an amino acid and opioid peptides are cleaved from precursor proteins.
All neurotransmitters undergo exocytosis from the presynaptic membrane and cross the synaptic cleft where they bind to their specific receptors. These receptors may be ligand-gated ion channels, such as ionotropic glutamate receptors, or G protein-coupled receptors, such as all sub-types of opioid receptor. Passage of ions through a ligand-gated ion channel alters the excitability of a neuron.
The action of neurotransmitters at GPCRs alters intracellular signaling pathways, with the specific ligand-gated being dependent on the G protein-coupled to the receptor.
Action Potentials
An action potential is a signal that transfers information along a neuron, while also triggering the release of a neurotransmitter at a synapse. In physical terms, an action potential is the rapid reversal of the resting membrane potential, engendered by the voltage-gated ion channels being opened and closed.
At rest, the cytosol of a neuron embodies a negative charge (polarized) concerning extracellular fluid, as a result of the distribution of ions across the cell membrane.
An action potential is actuated when the voltage-gated Na+ channels (Nav channels) open, permitting an influx of Na+ down its concentration gradient. This causes cell membrane depolarization beyond the threshold for action potential initiation. As inactivation occurs among Nav channels, inhibiting the flow of Na+, voltage-gated potassium channels (Kv channels) open, permitting the efflux of K+.
This triggers the cell membrane to undergo repolarization, as the balance of ion movement across the membrane causes the cytosol to take on a more negative charge than extracellular fluid. When Kv channels are open, cell membrane permeability to K+ is high, while Nav channels remain inactivated, meaning permeability to Na+ is low. This causes a period of hyperpolarization until Kv channels close and a re-establishment of the resting membrane potential.
The proliferation of an action potential transpires as a wave of depolarization that advances along an axon. When depolarization of an area of the membrane occurs, it opens adjacent Nav channels, which subsequently permit that section of the membrane to undergo depolarization. The inactivation of Nav channels in the preceding section of the membrane makes certain that an action potential moves in a singular direction along an axon.
Certain axons in the central nervous system are contained within a sheath, composed of myelin, which operates as an electrical insulator. Nav and Kv channels are localized on gaps in the myelin sheath, referred to as nodes of Ranvier. Myelination enhances the speed of action potential propagation as the action potential essentially ‘hops’ along an axon, taking place only at nodes of Ranvier in an operation referred to as saltatory conduction.
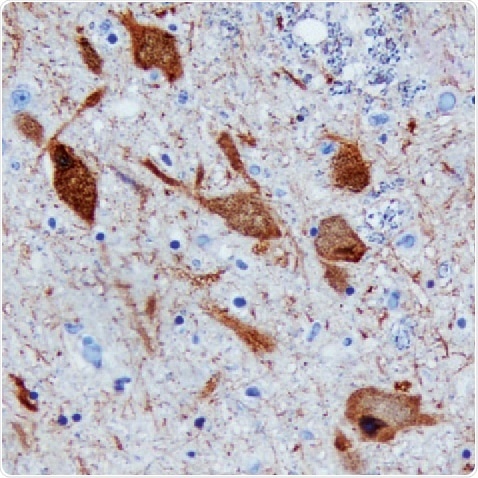
Figure 2. DDC in Human Brain. Detected in immersion-fixed paraffin-embedded sections of the human brain (substantia nigra) using a Goat Anti-Human/ Mouse/Rat DDC Antigen Affinity-Purified Polyclonal Antibody (R&D Systems). The tissue was stained using the Anti-Goat HRP-DAB Cell & Tissue Staining Kit (R&D Systems; brown) and counterstained with hematoxylin (blue. Tocris). Specific staining was localized to the neuronal cytoplasm.
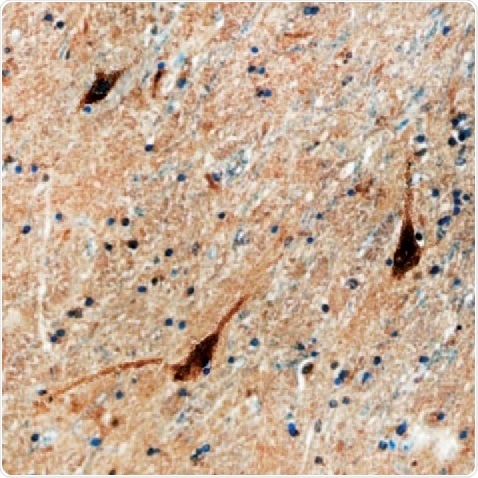
Figure 3. D1R in Human Brain. D1R detected in immersion fixed paraffin-embedded sections of human brain (caudate nucleus) using a Mouse Anti-Human Dopamine D1R Monoclonal Antibody (R&D Systems). The tissue was stained using the Anti-Mouse HRP-DAB Cell & Tissue Staining Kit (R&D Systems; brown) and counterstained with hematoxylin (blue. Tocris). Specific staining was localized to the neuronal cytoplasm.
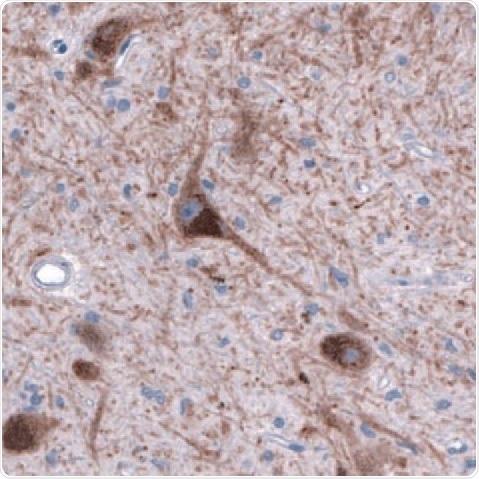
Figure 4. DAT1 in Human Brain. DAT1 detected in immersion-fixed sections of human brain (substantia nigra) using a Mouse Anti-Human/Mouse/Rat DAT1 Monoclonal Antibody (Novus Biologicals). The tissue was stained using HRP and DAB (brown) and counterstained with hematoxylin (blue. Tocris). Specific staining was localized to the dopamine neuron nuclei and fibers.
Further Reading and Featured Publications
Dopaminergic Transmission
- Beaulieu et al. (2015) Dopamine receptors – IUPHAR Review 13. Br J Pharmacol. 172, 1.
- Bertler & Rosengren (1959) Occurrence and distribution of dopamine in brain and other tissues. Experientia. 15, 10.
- Eisenhofer et al. (2004) Catecholamine metabolism: a contemporary view with implications for physiology and medicine. Pharmacol Rev. 56, 331.
- Kebabian & Calne (1979) Multiple receptors for dopamine. Nature. 277, 93.
- Laverty & Taylor (1970) Effects of intraventricular 2,4,5-trihydroxyphenylethylamine (6-hydroxydopamine) on rat behavior and brain catecholamine metabolism. Br J Pharmacol. 40, 836.
- Marsden (2006) Dopamine: the rewarding years. Br J Pharmacol. 147, S136.
Glutamatergic Transmission
- Anderson & Swanson (2000) Astrocyte glutamate transport: review of properties, regulation, and physiological functions. Glia. 32, 1.
- Curtis & Watkins (1965) The pharmacology of amino acids related to gamma-aminobutyric acid. Pharmacol Rev. 17, 347.
- MacDermott et al. (1986) NMDA-receptor activation increases cytoplasmic calcium concentration in cultured spinal cord neurones. Nature. 321, 519.
- McCulloch et al. (1974) The differential sensitivity of spinal interneurones and Renshaw cells to Kainate and N-methyl-D-aspartate. Exp Brain Res. 21, 515.
- Nakanishi (1992) Molecular diversity of glutamate receptors and implications for brain function. Science. 258, 597.
- Nicoletti et al. (2011) Metabotropic glutamate receptors: from the workbench to the bedside. Neuropharmacology. 60, 1017.
- Traynelis et al. (2010) Glutamate receptor ion channels: structure, regulation and function. Pharmacol Rev. 62, 405.
- Watkins & Jane (2006) The glutamate story. Br J Pharmacol. 147, S100.
Opioid Peptide Transmission
- Feng et al. (2012) Current research on opioid receptor function. Curr Drug Targets. 13, 230.
- Kelly (2013) Efficacy and ligand bias at the μ-opioid receptor. Br J Pharmacol. 169, 1430.
- Noble et al. (2015) The opioid receptors as targets for drug abuse medication. Br J Pharmacol. 172, 3964.
- Schwarzer (2009) 30 years of dynorphins – new insights on their functions in neuropsychiatric diseases. Pharmacol Ther. 123, 353.
- Toll et al. (2016) Nociceptin/Orphanin FQ receptor structure, signaling, ligands, functions, and interactions with opioid systems. Pharmacol Rev. 68, 419.
Serotonergic Transmission
- Bradley et al. (1986) Proposals for the classification and nomenclature of functional receptors for 5-hydroxytryptamine. Neuropharmacology. 25, 563.
- Blakely et al. (1994) Molecular physiology of norepinephrine and serotonin transporters. J Exp Biol. 196, 263.
- Hannon & Hoyer (2008) Molecular biology of 5-HT receptors. Behav Brain Res. 195, 198.
- Twarog & Page (1953) Serotonin content of some mammalian tissues and urine and a method for its determination. Am J Physiol. 175, 157.
- Yohn et al. (2017) The role of 5-HT receptors in depression. Mol Brain. 10, 28.
Chemogenetics
- Campbell & Marchant (2018) The use of chemogenetics in behavioural neuroscience: receptor variants, targeting approaches and caveats. Br J Pharmacol. 175, 994.
- Sternson & Roth (2014) Chemogenetic tools to interrogate brain functions. Annu Rev Neurol. 37, 387.
- Vardy et al. (2016) A new DREADD facilitates the multiplex chemogenetic interrogation of behavior. Neuron. 86, 936.
Depression
- Carr et al. (2010) Antidepressant-like effects of kappa-opioid receptor antagonists in Wistar Kyoto rats. Neuropsychopharmacology. 35, 752.
- McLaughlin et al. (2006a) Prior activation of kappa opioid receptors by U50,488 mimics repeated forced swim stress to potentiate cocaine place preference conditioning. Neuropsychopharmacology. 31, 787.
- McLaughlin et al. (2006b) Social defeat stress-induced behavioral responses are mediated by the endogenous kappa opioid system. Neuropsychopharmacology. 31, 1241.
- Pare (1992) The performance of WKY rats on three tests of emotional behavior. Physiol Behav. 51, 1051.
- Pare (1994) Openfield, learned helplessness, conditioned defensive burying, and forced-swim tests in WKY rats. Physiol Behav. 55, 433.
- Pearson et al. (2006) Identifying genes in monoamine nuclei that may determine stress vulnerability and depressive behavior in Wister-Kyoto rats. Neuropsychopharmacology. 31, 2449.
- Pringle et al. (2011) A cognitive neuropsychological model of antidepressant drug action. Prog Neuropyschopharmacol Biol Psychiatry. 35, 1586.
- Stuart et al. (2013) A translational rodent assay of affective biases in depression and antidepressant therapy. Neuropsychopharmacology. 38, 1625.
Ketamine Metabolites in Depression
- Berman et al. (2000) Antidepressant effects of ketamine in depressed patients. Biol Psychiatry. 47, 351.
- Holtman et al. (2008) Effects of norketamine enantiomers in rodent models of persistent pain. Pharmacol Biochem Behav. 90, 676.
- Zanos et al. (2016) NMDAR inhibition-independent antidepressant actions of ketamine metabolites. Nature. 533, 481.
- Zarate et al. (2006) A randomized trial of an N-methyl-D-aspartate antagonist in treatment-resistant major depression. Arch Gen Pyschiatry. 62, 856.
Addiction
- Baler & Volkow (2006) Drug addiction: the neurobiology of disrupted self-control. Trends Mol Med. 12, 559.
- Ferraro et al. (2010a) A novel mechanism of cocaine to enhance dopamine D2-like receptor mediated neurochemical and behavioral effects. An in vivo and in vitro study. Neuropsychopharmacology. 37, 1856.
- Ferraro et al. (2010b) Nanomolar concentrations of cocaine enhance D2-like agonist-induced inhibition of K+-evoked [3H]-dopamine efflux from rat striatal synaptosomes: a novel action of cocaine. J Neural Transm. 117, 593.
- Marchant et al. (2010) Medial dorsal hypothalamus mediated the inhibition of reward seeking after extinction. J Neurosci. 30, 14102.
- Rice & Cragg (2008) Dopamine spillover after quantal release: rethinking dopamine transmission in the nigrostriatal pathway. Brain Res Rev. 58, 303.
Epilepsy
- Goldman & Coulter (2013) Mechanisms of epileptogenesis: a convergence on neuronal circuit dysfunction. Nat Rev Neurosci. 14, 337.
- Klatte et al. (2013) Impaired D-serine-mediated cotransmission mediates cognitive dysfunction in epilepsy. J Neurosci. 33, 13066.
About Tocris Bioscience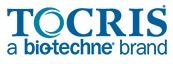
Tocris Bioscience is your trusted supplier of high-performance life science reagents, including receptor agonists & antagonists, enzyme inhibitors, ion channel modulators, fluorescent probes & dyes, and compound libraries. Our catalog consists of over 4,500 research tools, covering over 400 protein targets enabling you to investigate and modulate the activity of numerous signaling pathways and physiological processes.
We have been working with scientists for over 30 years to provide the life science community with research standards, as well as novel and innovative research tools. We understand the need for researchers to trust their research reagents, which is why we are committed to supplying our customers with the highest quality products available, so you can publish with confidence.
Tocris is part of the protein sciences division of Bio-Techne, which also includes the best in class brands R&D Systems, Novus Biologicals, ProteinSimple, and Advanced Cell Diagnostics. Bio-Techne has united these brands to provide researchers with a full portfolio of research reagents, assays, and protein platforms. For more information on Bio-Techne and its brands, please visit bio-techne.com.
Sponsored Content Policy: News-Medical.net publishes articles and related content that may be derived from sources where we have existing commercial relationships, provided such content adds value to the core editorial ethos of News-Medical.Net which is to educate and inform site visitors interested in medical research, science, medical devices, and treatments.