Neurodegeneration refers to the progressive death of neurons in the brain, leading to a loss of structure and function. The three most significant disorders that develop following neurodegeneration are Alzheimer’s, Parkinson’s, and Huntington’s disease.
Neurodegenerative Disease
Each disorder displays distinctive symptoms in the early stages of these diseases. A decline in cognitive functioning is characteristic of Alzheimer’s, diminished motor control is often linked with the early stages of Parkinson’s disease, while behavioral changes can be a symptom signifying Huntington’s disease.
Aging - An Important Factor
As we grow older, we become increasingly susceptible to these conditions, and this is thought to be linked to the heightened vulnerability of specific neuronal populations within defined areas of the brain.
It should be noted that around one-tenth of neurons die as a result of ‘healthy’, non-pathological aging. In fact, the protein aggregates which are considered synonymous with Alzheimer’s disease have also been detected in asymptomatic patients.
As a population gets older, the incidence rate of neurodegeneration is likely to show growth. Unfortunately, effective treatments to stop the progression of neurodegenerative disorders are conspicuously lacking, with no robust candidates currently insight.
Alzheimer’s disease is the most commonly diagnosed neurodegenerative disease globally. In 2010, it was estimated that 35.6 million people were affected, with these figures projected to almost double every two decades*, in line with an aging population.
Cause of Neuronal Cell Death In Neurodegenerative
At present, the processes that cause neuronal cell death in neurodegenerative diseases are poorly understood. However, it is thought that neurodegenerative diseases share common cellular and molecular mechanisms.
Each of the three diseases mentioned above is characterized by dysregulation of protein synthesis, degradation, and transport, alongside hallmark features, such as protein misfolding, accumulation into aggregates, and inclusion body formation.
A newly identified mechanism, which is relevant to all neurodegenerative diseases, indicates that cell death may come about through a build-up of misfolded proteins in the brain that over-stimulate a natural defensive mechanism – the unfolded protein response – halting the production of new proteins.
This causes the neuron to become starved of the proteins it requires for regular function and initiates cell death. In current studies, researchers are tackling this mechanism by attempting to inhibit the ‘off’ switch, potentially offering a turning point for the treatment of all neurodegenerative diseases.
Neurons’ Protective Machinery
Neurons have machinery that protects against the accumulation of misfolded and aggregated proteins. If chaperone proteins are unsuccessful in bringing about proper folding, abnormal proteins can be targeted for degradation by attachment of polyubiquitin and targeting to the proteasome for degradation.
Likewise, it is known that the autophagy/lysosomal pathways arbitrate between neuronal survival and death. However, when these processes are compromised, they can play pivotal roles in the pathogenesis of neurodegenerative diseases.
Genetic Components of Neurodegenerative Diseases
Many neurodegenerative diseases have an apparent genetic component. Huntington’s disease is a well-characterized polyglutamine disorder, in which a recurrence of the CAG sequence – which encodes the amino acid glutamine – produces a polyglutamine tract.
These additional glutamine residues prompt irregular protein folding and change protein function, which is lethal to the cell. Alzheimer’s and Parkinson’s disease also have genetic components, with genetic analysis recognizing various genes linked with each disorder, including ApoE4, PINK1, and LRRK2.
A significant example of the genetic component of Alzheimer’s can clearly be seen in people with Down Syndrome who possess the third copy of chromosome 21. Chromosome 21 is also the home of the gene that is linked with the production of the toxic amyloid β plaques in Alzheimer’s disease.
Almost every person with Down Syndrome who has this extra gene copy displays Alzheimer’s disease by 40 years of age.
Oxidative Stress
Another major characteristic that lies beneath neurodegenerative diseases is oxidative stress. Evidence of reactive oxygen species that are deadly to cells has been identified in affected brain regions of Alzheimer’s and Parkinson’s disease patients.
Oxidative stress not only causes harm to cells but can also induce programmed cell death, leading to neurodegeneration. However, it is not known whether the prevalent neuronal cell death witnessed in neurodegenerative diseases is a result of oxidative stress, or is coincident with it. As such, approaches to therapeutically impede oxidative stress may help decrease cell death.
Although neurodegenerative diseases display similarities in the cellular events that take place during the course of the disease, there are fundamental differences between each disorder that demand individual therapeutic approaches.
An example of where this is apparent is in the effects of L-DOPA. This drug eases motor symptoms in Parkinson’s disease, yet in Huntington’s disease, it aggravates motor dysfunction, even though the two diseases share a common cause of protein misfolding.
Further Reading
Please refer to the list of recommended papers for more information.
Alzheimer’s Disease
- De Strooper et al (2010) The secretases: enzymes with therapeutic potential in Alzheimer disease. Nat. Rev. Neurol. 6 99
- Eckert et al (2012) Mitochondrial dysfunction-a pharmacological target in Alzheimer’s disease. Mol. Neurobiol. 46 136
- Francis et al (1999) The cholinergic hypothesis of Alzheimer’s disease: a review of progress. J. Neurol. Neurosurg. Psychiatry
- 66 137
- Geldenhuys et al (2011) Role of serotonin in Alzheimer’s disease: a new therapeutic target? CNS Drugs 25 765
- Goedert et al (1991) Tau proteins and neurofibrillary degeneration. Brain Pathol. 1 279
- Goncalves et al (2013) Amyloid β inhibits retinoic acid synthesis exacerbating Alzheimer disease pathology which can be attenuated by an retinoic acid receptor α agonist. Eur. J. Neurosci. 37 1182
- Hynd et al (2004) Glutamate-mediated excitotoxicity and neurodegeneration in Alzheimer’s disease. Neurochem. Int. 45 583
- LaFerla et al (2007) Intracellular amyloid-β in Alzheimer’s disease. Nat. Rev. Neurosci. 8 499
- Massoud and Gauthier (2010) Update on the pharmacological treatment of Alzheimer’s disease. Curr. Neuropharmacol. 8 69
- Medhurst et al (2009) Characterization of histamine H3 receptors in Alzheimer’s Disease brain and amyloid over-expressing TASTPM mice. Br. J. Pharmacol. 157 130
- Noble et al (2013) The importance of tau phosphorylation for neurodegenerative diseases. Front Neurol. 4 83
- Ramírez et al (2005) Prevention of Alzheimer’s disease pathology by cannabinoids: neuroprotection mediated by blockade of microglial activation. J. Neurosci. 25 1904
- Salomone et al (2012) New pharmacological strategies for treatment of Alzheimer’s disease: focus on disease modifying drugs. Br. J. Clin. Pharmacol. 73 504
- Savelieff et al (2013) Untangling amyloid-β, tau, and metals in Alzheimer’s disease. ACS Chem. Biol. 8 856
- Selenica et al (2007) Efficacy of small-molecule glycogen synthase kinase-3 inhibitors in the postnatal rat model of tau hyperphosphorylation. Br. J. Pharmacol. 152 959
- Serý et al (2013) Molecular mechanisms of neuropathological changes in Alzheimer’s disease: a review. Folia Neuropathol. 51 1
- Siedlak et al (2009) Chronic antioxidant therapy reduces oxidative stress in a mouse model of Alzheimer’s disease. Free Radic. Res. 43 156
Parkinson’s Disease
- Abou-Sleiman et al (2006) Expanding insights of mitochondrial dysfunction in Parkinson’s disease. Nat. Rev. Neurosci. 7 207
- Exner et al (2012) Mitochondrial dysfunction in Parkinson’s disease: molecular mechanisms and pathophysiological consequences. EMBO J. 31 3038
- Henchcliffe and Beal (2008) Mitochondrial biology and oxidative stress in Parkinson’s disease pathogenesis. Nat. Clin. Pract. Neurol. 4 600
- Huot et al (2013) The pharmacology of L-DOPA-induced dyskinesia in Parkinson’s disease. Pharmacol. Rev. 65 171
- Lanciego et al (2012) Functional neuroanatomy of the basal ganglia. Cold Spring Harb. Perspect. Med. 2 a009621
- Lee et al (2012) Apoptosis signal-regulating kinase 1 mediates MPTP toxicity and regulates glial activation. PLoS One. 7 e29935
- Lee et al (2013) Parthanatos mediates AIMP2-activated age-dependent dopaminergic neuronal loss. Nat. Neurosci. 16 1392
- Lundblad et al (2012) Impaired neurotransmission caused by overexpression of α‑synuclein in nigral dopamine neurons. Proc. Natl. Acad. Sci. U. S. A. 109 3213
- McNaught et al (2003) Altered proteasomal function in sporadic Parkinson’s disease. Exp. Neurol. 179 38
- Surmeier and Schumacker (2013) Calcium, bioenergetics, and neuronal vulnerability in Parkinson’s disease. J. Biol. Chem. 288 10736
- Venderova and Park (2012) Programmed cell death in Parkinson’s disease. Cold Spring Harb. Perspect. Med. 2 a009365
Huntington’s Disease
- Arraste et al (2004) Inclusion body formation reduces levels of mutant huntingtin and the risk of neuronal death. Nature 431 805
- Bano et al (2011) Neurodegenerative processes in Huntington’s disease. Cell Death Dis. 2 e228
- Beal and Ferrante (2004) Experimental therapeutics in transgenic mouse models of Huntington’s disease. Nat. Rev. Neurosci. 5 373
- Crook and Housman (2011) Huntington’s disease: can mice lead the way to treatment? Neuron 69 423
- Frank and Jankovic (2010) Advances in the pharmacological management of Huntington’s disease. Drugs 70 56
- Kaplan and Stockwell (2012) Therapeutic approaches to preventing cell death in Huntington disease. Prog. Neurobiol. 99 262
- Labbadia and Morimoto (2013) Huntington’s disease: underlying molecular mechanisms and emerging concepts. Trends Biochem. Sci. 38 378
- Landles and Bates (2004) Huntingtin and the molecular pathogenesis of Huntington’s disease. Fourth in Molecular Medicine Review Series. EMBO Rep. 5 958
- Perrier and Peschanski (2013) How can human pluripotent stem cells help decipher and cure Huntington’s disease? Cell Stem Cell 11 153
- Ravikumar et al (2004) Inhibition of mTOR induces autophagy and reduces toxicity of polyglutamine expansions in fly and mouse models of Huntington disease. Nat. Genet. 36 585
- Ross and Tabrizi (2011) Huntington’s disease: from molecular pathogenesis to clinical treatment. Lancet Neurol. 10 83
About Tocris Bioscience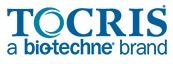
Tocris Bioscience is your trusted supplier of high-performance life science reagents, including receptor agonists & antagonists, enzyme inhibitors, ion channel modulators, fluorescent probes & dyes, and compound libraries. Our catalog consists of over 4,500 research tools, covering over 400 protein targets enabling you to investigate and modulate the activity of numerous signaling pathways and physiological processes.
We have been working with scientists for over 30 years to provide the life science community with research standards, as well as novel and innovative research tools. We understand the need for researchers to trust their research reagents, which is why we are committed to supplying our customers with the highest quality products available, so you can publish with confidence.
Tocris is part of the protein sciences division of Bio-Techne, which also includes the best in class brands R&D Systems, Novus Biologicals, ProteinSimple, and Advanced Cell Diagnostics. Bio-Techne has united these brands to provide researchers with a full portfolio of research reagents, assays, and protein platforms. For more information on Bio-Techne and its brands, please visit bio-techne.com.
Sponsored Content Policy: News-Medical.net publishes articles and related content that may be derived from sources where we have existing commercial relationships, provided such content adds value to the core editorial ethos of News-Medical.Net which is to educate and inform site visitors interested in medical research, science, medical devices, and treatments.