Healthy tissues can be distinguished from diseased tissues with the help of a highly detailed image of the organs provided by non-invasive diagnostics that make use of contrast agents. The functioning of contrast agents varies with the diagnostic procedure for which they are used.
For instance, when used for magnetic resonance imaging (MRI), the contrast media function using the magnetic field produced by charged particles, such as electrons and protons, while radio contrast agents (RCA) modify the X-ray absorption of the organ being radiographed in line with the surrounding tissue.
Unlike conventional X-ray imaging, MRI does not use ionizing radiation. The working principle of MRI is the same as NMR spectroscopy, leveraging the emission and absorption energy within the radio frequency range. In MRI, the nucleus of the hydrogen atom plays a significant role due to its intrinsic angular momentum that causes magnetic momentum and the frequency of its occurrence in protein molecules or water or fat in the organism.
A phenomenon called relaxation occurs, where a short, high-frequency pulse alters the spin orientation of the protons, prior to the spin, followed by returning to the original state by emitting energy that was absorbed earlier when the signal is switched off.
A highly-sensitive detector coil captures the emitted signals, and the recorded signals are in high-contrast cross sections. In order to enhance the quality of imaging, paramagnetic contrast agents are used, in which unpaired electrons are present in the outer shell, and hence, a magnetic momentum is also present.
The magnetic momentum of electrons, is 657-times more than that of protons, and therefore, they have a greater influence on protons. The interaction between the contrast agent’s electron spin and the neighboring hydrogen nuclei directly alters the proton density in the tissue, and indirectly affects the local magnetic field.
In addition to paramagnetic elements, iron and manganese, another prominent MRI contrast agent is lanthanide gadolinium, with seven unpaired electrons in its outer shell. A major limitation in the use of lanthanide gadolinium is its innate toxicity because of which it can only be used in its water-soluble chelate complex form (Figure 1), and not in the free ionic state (Gd3+).
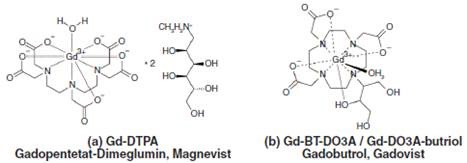
Figure 1. Chemical structures of two gadolinium chelates typically used in MRI, (a) Magnevist with linear and (b) Gadovist with macrocyclic polyaminopolycarboxylic acid ligands.
More specifically, the derivatives of diethylenetriaminepentaacetic acids (DTPA) are known to be highly effective contrast-enhancing agents in MRI. The in vivo and in vitro stability of gadolinium contrast agents, which are mostly delivered intravenously, are exceptionally high.
The administered gadolinium has a half-life of 2 hours roughly, and as a result, it remains unaltered when it is expelled via the kidney-urine path and eventually reaches the wastewater. In order to enhance the settling behavior of the colloidal material that is suspended and dissolved in the wastewater, flocculation is carried out.
As a result of flocculation through the addition of trivalent metal ions like Al3+ and Fe3+, there is a contest between the Gd3+ ions and trivalent metal ions for the organic ligands.
The recomplexing or transmetallation that occurs in MRI contrast agents and the subsequent release of toxic Gd3+ ions is brought about by the introduction of trivalent metal salts based on the thermodynamic complex stability. The exchange of metals that occurs in gadolinium chelate Magnevist due to iron flocculation is shown in Figure 2.
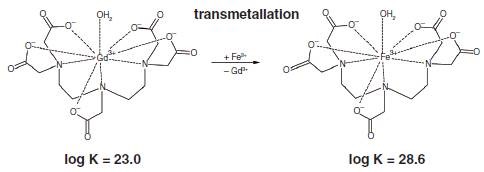
Figure 2. Displacement of gadolinium from Magnevist by addition of Fe3+. The thermodynamic stability constants are given under the chelate complexes.
This article discusses the determination of the amount of toxic Gd3+ ions released by Fe3+ flocculation carried out in the wastewater treatment plants, using the ion chromatography followed by inductively coupled plasma mass spectrometry (ICP-MS). Here, the test compounds, Gadovist and Magnevist, are taken in their aqueous solution form for examining the effect of bench-scale flocculation.
Experimental
Differentiating between various oxidation stages of metal ions (speciation) and between bound and free ions can be done with IC–ICP-MS. This capability makes it an ideal method for examining the impact of adding Fe3+ for flocculation on the stability of gadolinium chelates.
The Metrosep A Supp 3 - 250/4.6 anion separation column was used to separate the contrast agents, and a Nucleosil 5 SA - 125/4.0 cation separation column with a 2-hydroxyisobutyric acid eluent was used for analyzing the Gd3+ ions that were displaced.
The organic content in the eluent was high, resulting in carbon deposition on the skimmer and sampler cones, which, in turn, affected the inline coupling between the IC and ICP-MS. These deposits were removed by treating the IC fractions (4.5mL) with 2.5mL of concentrated nitric acid prior to the ICP-MS analysis.
The combustion of organic compounds in ICP plasma and their oxidation were enhanced by earlier acidification. The MRI contrast agents were aqueous solutions of Gadovist and Magnevist, in which the concentration of gadolinium was 1mg/L. The stability of the gadolinium contrast agents were evaluated for 5, 10 and 20mg/L concentrations of Fe3+.
The instrumentation setup for the IC–ICP-MS analysis consisted of 850 Professional IC (Metrohm) and a VG PQ ExCell ICP-MS. Table 1 shows the ICP-MS determination parameters, and Figures 3 and 4 present the IC parameters in the form of chromatograms.
Table 1. ICP-MS determination parameters
Mode
|
No gas
|
Power
|
1200W
|
Nebulizer
|
Concentric
Flow rate – 1mL/min
|
Flows
|
Plasma gas
|
Ar, 13L/min
|
Auxiliary gas
|
Ar, 1L/min
|
Nebulizer gas
|
Ar, 1L/min
|
Detection
|
156Gd, 158Gd, 160Gd
|
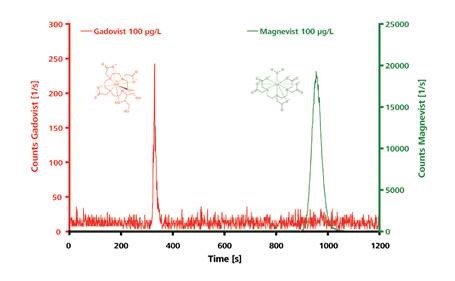
Figure 3. IC–ICP-MS chromatograms for the polar and electrically neutral Gadovist and the ionic Magnevist (both 100µg/L). Separation of the intact chelates was carried out on a Metrosep A Supp 3 - 250/4.0. Eluent: 6.8mmol/L NaHCO3, 7.2mmol/L Na2CO3, 1.0mL/min; column temperature: 25°C; sample volume: 100µL.
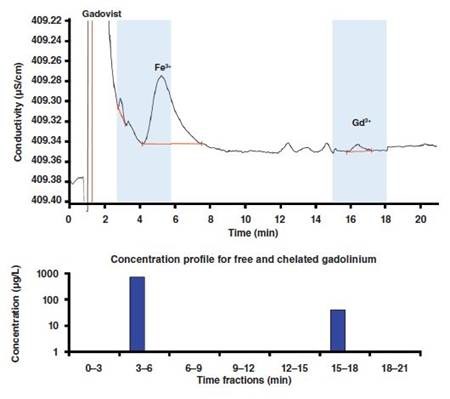
Figure 4. Concentration profile for free and chelated gadolinium in a Gadovist sample to which Fe3+ was added up to a final concentration of 5mg/L. Column: Nucleosil 5 SA - 125/4.0; eluent: 10mmol/L 2-hydroxyisobutyric acid,2mmol/L ethylenediamine, 1.5mL/min; column temperature: 30°C; sample volume: 100µL.
Results
Two distinctly separated peaks for Gadovist and Magnevist are produced on the anion exchange column as a result of the IC-ICP-MS analysis. Elution of Gadovist occurs within a few minutes because it is a polar, uncharged compound, while Magnevist is retained for a longer duration and elutes at a later stage due to its double negative charge.
The Nucleosil cation separation column is used for determining the concentrations of the participating Gd3+ and Fe3+ present in the polyaminopolycarboxylate ligands and the degree of transmetallation. Fractionation method was followed for the whole ion chromatographic separation process in order to obtain a final concentration of 5mg/L by adding Fe3+ ions to a Gadovist solution with a Gd concentration of 1mg/L. The concentration profile depicted in Figure 4 may be used for the released Gd3+ ions.
The early eluting fraction is found to contain Gadovist, since it elutes with the solvent peak due to its electrically neutral nature. This implies that the chelated and free metal cations can be separated on an on-line IC–ICP-MS coupling. The gadolinium displacement for the contrast agents Gadovist and Magnevist is shown in Figure 5. It can be seen from the figure that there is a proportional increase in the gadolinium displacement as the concentration of Fe3+ increases. Magnevist exhibits this behavior more prominently.
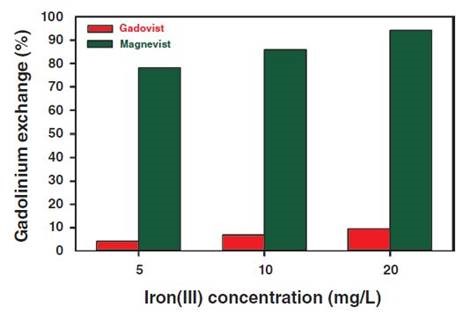
Figure 5. Gadolinium displacement determined from Gadovist and Magnevist as a function of the final Fe3+ concentration.
Recomplexing of Magnevist [log K = 23.0; (3)] to 80% is possible even with 5mg/L Fe3+ because the iron(III)- DTPA complex [log K = 28.6; (3)] has a higher thermodynamic stability. The percentage of iron chelate complex formed is even more when Fe3+ concentration is 20mg/L, reaching over 90%.
On the contrary, very small amount of the released Gd3+ ions were from Gadovist, even at higher concentration of Fe3+ because transmetallation is greatly avoided. This is due to the presence of spherically arranged crown-ether-like polyaminopolycarboxylic acid ligand around the gadolinium so that Fe3+ ions are not allowed to compete with the complex center of the Gadovist.
In case of Magnevist, the shielding of the central atom by the linear DTPA ligands is not strong enough to prevent transmetallation, and therefore, a stable iron(III) complex is formed due to the substitution of gadolinium by iron.
Conclusion
Highly reliable, sensitive and fast speciation analysis of free and chelated gadolinium compounds in wastewater can be performed when ion chromatography (IC) is used in conjunction with inductively coupled plasma mass spectrometry (IC–ICP-MS).
This analysis method provides critical information pertaining to the supply, deterioration and fate of the contrast agents in wastewater without any expensive sample preparation steps. Further, detection of gadolinium-based compounds in biological matrixes like blood or urine can be effectively carried out by the IC-ICP-MS method.
References
- J. Künnemeyer et al., Environ. Sci. Technol., 43, 2884–2890 (2009).
- K. Kümmerer, Pharmaceuticals in the environment – sources, fate, effects, and risks, K. Kümmerer (Ed.), Springer Verlag, Berlin, 3rd ed., 521 pp. (2008).
- J. Künnemeyer et al., Anal. Chem., 81, 3600–3607 (2009).
- A. Bianchi et al., Coordination Chemistry Reviews, 204, 309–393 (2000).
Acknowledgements
Produced from materials authored by Peter Pfundstein1, Christian Martin2, Wolfgang Schulz3, Ruth Katinka Meike4, Andrea Wille4, Thomas Moritz4, Alfred Steinbach4 and Dirk Flottmann1 from:
1Aalen University, Aalen, Germany,
2Leibniz Center for Tropical Marine Ecology (ZMT), Bremen, Germany,
3Zweckverband Landeswasserversorgung, Betriebs- und Forschungslaboratorium, Langenau, Germany,
4Metrohm International Headquarters, Herisau, Switzerland.
About Metrohm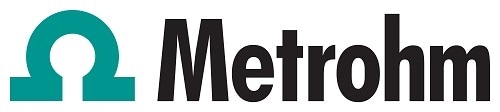
At Metrohm is one of the world’s most trusted manufacturers of high-precision instruments for chemical analysis. Metrohm was founded in 1943 by engineer Bertold Suhner in Herisau, Switzerland. Today, Metrohm is represented in 120 countries by subsidiaries and exclusive distributors. The global Metrohm Group also includes the Dutch companies Metrohm Applikon and Metrohm Autolab, manufacturers of online analyzers and instruments for electrochemical research, respectively. Recently, the Metrohm Group was joined by Metrohm Raman, a leading manufacturer of handheld Raman spectrometers.
Metrohm is the global market leader in analytical instruments for titration. Instruments for ion chromatography, voltammetry, conductivity, and stability measurement make the Metrohm portfolio for ion analysis complete. Instruments for Near-infrared and Raman spectroscopy are another, strongly growing segment of the Metrohm portfolio.
Metrohm is a problem solver, both in the laboratory and within the industrial process. To this end, the company offers their customers complete solutions, including dedicated analytical instrumentation as well as comprehensive application know-how. More than 30% of the company’s employees at the Metrohm international headquarters in Herisau work in R&D.
Metrohm has been owned 100% by the non-profit Metrohm Foundation since 1982. The Metrohm Foundation, which does not exert any influence on the company’s business operations, sponsors gifted students in the natural sciences, supports charitable and philanthropic purposes and, above all, ensures the independence of the company.
Sponsored Content Policy: News-Medical.net publishes articles and related content that may be derived from sources where we have existing commercial relationships, provided such content adds value to the core editorial ethos of News-Medical.Net which is to educate and inform site visitors interested in medical research, science, medical devices and treatments.